Will Genome Modifications Define Next-Gen AAV Vectors?
A conversation with Arun Srivastava, Ph.D., University of Florida College of Medicine
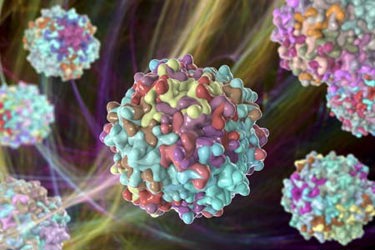
Adeno-associated viruses (AAV) are notoriously imperfect therapeutic carriers, and experts predict that the next generation of AAV serotypes will include thoughtful, low-touch genetic modifications that transform their profiles for the better.
Arun Srivastava, Ph.D., a University of Florida College of Medicine professor and one of the pioneers of AAVs, has been speaking about what the next generation of genome-modified AAV vectors will look like, and he agreed to share some of his perspectives with us, so we sent him some questions. Here’s how he answered them.
Given the clinical successes of first-generation AAV vectors, what limitations motivated the pursuit of capsid-modified next-gen vectors?
I agree that whereas some of the FDA-approved first generation of AAV vectors have been quite successful:
- Luxturna for Leber congenital amaurosis,
- Zolgensma for spinal muscular atrophy,
- Hemgenix for hemophilia B, and
- Kebilidi for aromatic L-amino acid decarboxylase deficiency.
Others have been less so:
- Elevidys for Duchenne muscular dystrophy,
- Roctavian for hemophilia A, and
- Beqvez for hemophilia B (Beqvez has now been discontinued).
As I stated in my commentary,1 based on a comment from Dr. Jeffrey Bluestone at the end of his keynote lecture at the 2001 Annual Meeting of Gene (and Cell) Therapy, I surmised that since AAV evolved as a virus, and not as a vector, the first generation of AAV vectors composed of the naturally occurring capsids would be suboptimal. This assumption was based on the logic that the host immune system would not be able to distinguish between the naturally occurring AAV and the first generation of AAV vectors. In other words, AAV vectors had to be different from AAV as a virus to evade the host immune responses, both cellular and humoral.
I should also add that the use of exceedingly high doses of first-generation AAV vectors have also led to the deaths of 13 patients to date. 2,3
What role will first-gen AAV vectors have in new discovery? Are you predicting a total departure from unmodified capsids?
I am reasonably optimistic that focusing on the basic biology of AAV will guide us in designing AAV vectors that are safe, less immunogenic, and more efficient at lower doses. No, I am not predicting a total departure from unmodified capsids since the first generation of AAV vectors work quite well in organs that are immune-privileged, such as the eye and brain.
Also, as stated by the grandfather of AAV, the late Dr. Kenneth I. Berns, in his commentary,4 “the only component of the AAV genome required in the vector particle is the ITR, the sequence of which we have known since 1980. Yet, we still do not understand the details of its higher order structure or the full range of its biological properties,” the efficacy of even the first-gen AAV vectors will be significantly improved.
Between ITRs and capsid modifications, which area holds more immediate promise for enhancing efficacy and safety of AAVs?
Capsid and ITR modifications may not necessarily be mutually exclusive. A combination of the two may well be desirable. However, the time, effort, and resources that have been, and continue to be, expended in AAV capsid engineering have had diminishing returns. Several clinical trials with capsid-engineered AAV vectors have not been successful for the simple reason that extensive modifications in the capsid likely leads to creation of completely unknown novel antigenic epitopes, both T and B cell-specific, leading to increased immunogenicity in humans. 3 In this context, it is also important to note that, to a certain extent, evaluation of extensively capsid-engineered AAV vectors in preclinical studies in animal models, both small and large, has not been predictive of safety of these vectors since humans are not large mice or monkeys.
In other words, it may be advisable to keep the extent of capsid modifications to a minimum such that the vector is different from the virus and does not trigger the immune response – the Goldilocks solution!
Looking at the promise of lowering doses with modified AAV vectors, what analytical techniques and preclinical models help to determine the ideal therapeutic window?
Lowering the doses of modified AAV vectors, without compromising their therapeutic efficacy, will have the following two major consequences:
- First, the lower vector dose per patient will obviate the need for prophylactic immune-suppression that is frequently used in most, if not all, current AAV gene therapy trials and which has its own potential complications.
- And second, the lower vector dose per patient will also allow more patients to be treated than high vector doses that are currently being used. In other words, instead of treating one patient, 10, or maybe 100, patients could be treated, which would not only bring down the cost per patient but also reduce the vector production costs.
In my view, whereas the analytical techniques will most likely remain unchanged, I am now convinced more than ever that none of the preclinical animal models is a true surrogate for or a predictor of therapeutic efficacy in human diseases. Thus, our focus is now only on AAV serotype vectors that are capable of transducing primary human cells. For example, we have identified AAV3 vectors to be remarkably efficient in transducing primary human hepatocytes, 5,6 and AAV6 vectors for transducing primary human hematopoietic stem cells. 7,8 The hope and the expectation is that they will also be efficacious in clinical trials in humans, for only then we will be able to determine the ideal therapeutic window.
Describe the limitations in large-scale manufacturing and purification of these more complex, modified AAV vectors. How are these challenges being addressed?
In our experience thus far, large-scale manufacturing and purification of the several minimally modified AAV serotype vectors have not been challenging. The same is also true for the ITR-modified vectors, although I must acknowledge that whereas the titers of some are the same as those containing the unmodified ITRs, 9 some are reduced by about 50%.10 However, as I stated above, I am fairly confident that by pursuing the basic molecular biology underlying the mechanism of packaging of the AAV genome, we will eventually overcome this obstacle.
How do you envision the regulatory landscape evolving to accommodate the unique characteristics and potential benefits of these next-generation AAV gene therapies?
My hope is that given that the minimal changes in several AAV serotype capsids have led to improved transduction in animal models, both small and large,11-13 and in a Phase 1/2 clinical trial in humans, 14 these potential benefits would be viewed favorably by the regulatory agencies.
Increased transgene expression from ITR-modified vectors9,10 and the combination of both capsid- and ITR-modifications, which lead to at least additive, if not synergistic, effects in mice,15,16 provide further a basis for optimism that the use of these novel AAV serotype vectors will likely lead to significant performance improvements in humans at further reduced doses. By that, I mean lower immunogenicity, improved safety, and reduced vector production costs as well as cost per patient for gene therapy of a wide variety of diseases, ensuring translation to the clinic with higher probability of success, not only in the developed world but also in low- and middle-income countries, where most patients reside and are in desperate need of such therapies.
References:
- Srivastava A. Adeno-Associated Virus: The Naturally Occurring Virus Versus the Recombinant Vector. Hum Gene Ther. 2016;27(1):1-6.
- Srivastava A. AAV Vectors: Are They Safe? Hum Gene Ther. 2020;31(13-14):697-699.
- Srivastava A. Rationale and strategies for the development of safe and effective optimized AAV vectors for human gene therapy. Mol Ther Nucleic Acids. 2023;32:949-959.
- Berns KI. The Unusual Properties of the AAV Inverted Terminal Repeat. Hum Gene Ther. 2020;31(9-10):518-523.
- Vercauteren K, Hoffman BE, Zolotukhin I, Keeler GD, Xiao JW, Basner-Tschakarjan E, High KA, Ertl HC, Rice CM, Srivastava A, de Jong YP, Herzog RW. Superior In vivo Transduction of Human Hepatocytes Using Engineered AAV3 Capsid. Mol Ther. 2016;24(6):1042-1049.
- Brown HC, Doering CB, Herzog RW, Ling C, Markusic DM, Spencer HT, Srivastava A, Srivastava A. Development of a Clinical Candidate AAV3 Vector for Gene Therapy of Hemophilia B. Hum Gene Ther. 2020.
- Song L, Kauss MA, Kopin E, Chandra M, Ul-Hasan T, Miller E, Jayandharan GR, Rivers AE, Aslanidi GV, Ling C, Li B, Ma W, Li X, Andino LM, Zhong L, Tarantal AF, Yoder MC, Wong KK, Jr., Tan M, Chatterjee S, Srivastava A. Optimizing the transduction efficiency of capsid-modified AAV6 serotype vectors in primary human hematopoietic stem cells in vitro and in a xenograft mouse model in vivo. Cytotherapy. 2013;15(8):986-998.
- Ling C, Bhukhai K, Yin Z, Tan M, Yoder MC, Leboulch P, Payen E, Srivastava A. High-Efficiency Transduction of Primary Human Hematopoietic Stem/Progenitor Cells by AAV6 Vectors: Strategies for Overcoming Donor-Variation and Implications in Genome Editing. Sci Rep. 2016;6:35495.
- Lu Y, Ling C, Shoti J, Yang H, Nath A, Keeler GD, Qing K, Srivastava A. Enhanced transgene expression from single-stranded AAV vectors in human cells in vitro and in murine hepatocytes in vivo. Mol Ther Nucleic Acids. 2024;35(2):102196.
- Ling C, Wang Y, Lu Y, Wang L, Jayandharan GR, Aslanidi GV, Li B, Cheng B, Ma W, Lentz T, Ling C, Xiao X, Samulski RJ, Muzyczka N, Srivastava A. Enhanced transgene expression from recombinant single-stranded D-sequence-substituted adeno-associated virus vectors in human cell lines in vitro and in murine hepatocytes in vivo. J Virol. 2015;89(2):952-961.
- Zhong L, Li B, Mah CS, Govindasamy L, Agbandje-McKenna M, Cooper M, Herzog RW, Zolotukhin I, Warrington KH, Jr., Weigel-Van Aken KA, Hobbs JA, Zolotukhin S, Muzyczka N, Srivastava A. Next generation of adeno-associated virus 2 vectors: point mutations in tyrosines lead to high-efficiency transduction at lower doses. Proc Natl Acad Sci U S A. 2008;105(22):7827-7832.
- Li S, Ling C, Zhong L, Li M, Su Q, He R, Tang Q, Greiner DL, Shultz LD, Brehm MA, Flotte TR, Mueller C, Srivastava A, Gao G. Efficient and Targeted Transduction of Nonhuman Primate Liver With Systemically Delivered Optimized AAV3B Vectors. Mol Ther. 2015;23(12):1867-1876.
- Kumar SRP, Xie J, Hu S, Ko J, Huang Q, Brown HC, Srivastava A, Markusic DM, Doering CB, Spencer HT, Srivastava A, Gao G, Herzog RW. Coagulation factor IX gene transfer to non-human primates using engineered AAV3 capsid and hepatic optimized expression cassette. Mol Ther Methods Clin Dev. 2021;23:98-107.
- Guy J, Feuer WJ, Davis JL, Porciatti V, Gonzalez PJ, Koilkonda RD, Yuan H, Hauswirth WW, Lam BL. Gene Therapy for Leber Hereditary Optic Neuropathy: Low- and Medium-Dose Visual Results. Ophthalmology. 2017;124(11):1621-1634.
- Ling C, Li B, Ma W, Srivastava A. Development of Optimized AAV Serotype Vectors for High-Efficiency Transduction at Further Reduced Doses. Hum Gene Ther Methods. 2016;27(4):143-149.
- Shoti J, Qing K, Keeler GD, Duan D, Byrne BJ, Srivastava A. Development of capsid- and genome-modified optimized AAVrh74 vectors for muscle gene therapy. Mol Ther Methods Clin Dev. 2023;31:101147.
About The Expert:
Arun Srivastava, Ph.D., is a University of Florida College of Medicine professor in the pediatrics, molecular genetics, and microbiology departments, and the college’s Powell Gene Therapy Center. He was a founder of the of the first AAV gene therapy company, Avigen, in 1992, and later cofounded Lacerta Therapeutics, sAAVient Therapeutics, and nAAVigen Therapeutics. He serves on an NIH study section and the editorial boards of several academic journals. He has published more than 220 peer-reviewed articles, book chapters, reviews, and other articles. He received his Ph.D. from the Indian Institute of Science in Bangalore and completed postdoctoral training at Memorial Sloan-Kettering Cancer Center. He was recently elected as a Fellow of the American Society for the Advancement of Science.