Why Repeatability of Dosing Matters for Gene Delivery
By Jonathan Thon, Ph.D., Founder and CEO and Laura Goldberg, M.D., Ph.D., VP of R&D at STRM.BIO
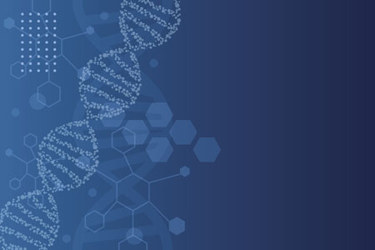
Gene therapies offer the potential to change lives in the most profound way. Sight can be restored. Hemophilia can be corrected. The progression of Duchenne muscular dystrophy can be slowed. These advances are celebrated, but invariably followed by questions about the durability of treatment. The benefit offered by a gene therapy can fade over time due to declining transgene expression. Will the therapeutic effect last a year, 10 years, 20 years, a lifetime? Wait and see. What is true is that the idea of “one-and-done” therapies is akin to the tail wagging the dog. Most “single” dose gene therapies are likely to require a second or third dose to achieve therapeutic benefit, and the number of diseases that can theoretically (let alone practically) be treated with a single dose represent a very small sliver of the genetic disorders, which society would like to cure.
The Challenge of Repeat Dosing
Muhuri et al, outlined the non-immunological and immunological processes that may limit or improve the durability of gene therapies and strategies that can be used to increase the duration of the therapeutic effect.1 Limiting factors include epigenetic processes such as transgene silencing, cell turnover in transduced cells, cellular stress, and innate and adaptive immune responses.
At the 2023 American Society of Cell and Gene Therapy annual meeting, several early-stage strategies designed to allow a second gene therapy dose were highlighted including the use of cancer2 and organ transplant3 drugs, and dosing with different, distinct AAV clades.4
In addition to durability challenges, vectors that can only be dosed once are likely to be insufficient for treating diseases where the gene therapy target may change over time. This is most notable in the oncologic space, where tumors are notorious for their ability to evolve and escape destruction by the immune system.5 In these cases, being able to re-dose patients with gene therapy constructs aimed at new targets would be essential for continued anti-cancer treatments. In vivo CAR-T cell generation could be particularly well-suited here. Repeat dosing of CAR-T constructs designed to attack new cancer targets in vivo could combat a changing tumor antigen landscape.
An inability to repeat dosing also means that, independent of durability, the first dose of a gene therapy construct must achieve enough correction to impart clinical benefit. For example, in sickle cell disease, it has been estimated that at least 20% correction may likely be required to reverse the sickle cell phenotype.6 If the first dose falls short of this clinically relevant threshold, the inability to repeat dose would render the gene therapy treatment ineffective. It also means that when that dose is insufficient, the patient may be left with fewer treatment options.
In addition to editing a threshold percentage of stem and progenitor cells before a sustainable clinical benefit is seen, some stem and progenitor cells won’t be targetable at all. In this case, it will be necessary to treat, rather than cure, the disease using regular doses that edit each new generation of shorter-lived non-stem cells.
If a gene therapy delivery vector does not possess immune privilege, the patient’s immune system may recognize it as an external threat and begin priming the system against the virus, with development of both humoral and T cell immunity.7 An immunogenic gene therapy delivery vector will thus act essentially as a vaccine against future doses of the same therapy. This repeat dosing conundrum is evident when viruses including adenoviral (AdV) and adeno-associated virus (AAV) vectors are used for the initial in vivo delivery of the therapy. An immune response to the vectors in the form of neutralizing antibodies can eliminate the option to re-dose the same therapy. The immune response can also cause side-effects, some of which can be serious.8,9
The Need for Gene Delivery Platforms Compatible with Repeat Dosing
Instead of retrofitting new components to existing technologies to make them work better (virus 4.0), we should be developing new gene delivery platforms from the start that are compatible with repeat dosing.
The current generation of synthetic particles has a better safety profile than viral vectors, although a small percentage of the population may mount an immune, inflammatory, or (very rarely) even an allergic response to the ingredients of some formulations.10 Nevertheless, synthetic particles exhibit better immune privilege than viral vectors for most individuals, permitting a limited number of repeat doses.11
Biologically derived, non-viral delivery approaches for gene therapy include extracellular vesicles (EVs), which are lipid-based particles that are naturally secreted by almost all cell types. The EV category includes exosomes which derive from inside cells and microvesicles (also called ectoparticles or microparticles), which bud from the cell surface membrane and thus would be expected to have the necessary immune privilege to enable repeat dosing if needed. An essential part of the intercellular communication network, EVs encapsulate RNAs, DNAs, and proteins and efficiently deliver this cargo into other cells—several orders of magnitude more efficiently than lipid nanoparticles (LNPs) and other synthetic particles.12 Exosomes and microvesicles recently entered development as delivery vehicles for gene therapy, especially for applications targeting hematopoietic stem and progenitor cells.13,14,15,16
The Repeatability Potential of Microvesicles
EVs are ideally situated to address the gene therapy challenge of durability through repeat dosing. Not all EVs are created equally, however, and cell source matters.
In one important study in which human embryonic kidney cell-derived EVs were administered to a non-human primate model, the EV circulation times decreased with repeated intravenous administration, raising the possibility of immune-mediated clearance, potentially due to the xenogenicity (Dreidonks et al).17
Conversely, there have been multiple studies showing minimal toxicity and immunogenicity of systemically administered EVs derived from various cell sources including human embryonic kidney cells. mesenchymal stem cells, and oligodendrocytes, with repeat dosing strategies eliciting no detectable adverse immune responses.18,19,20,21,22 Interestingly, when AAV was enveloped by or associated with EVs, the EV-associated AAV was shielded from neutralizing antibodies in vivo.23 These data underscore the potential of select EV-based platforms to evade the immune system and be particularly well-suited for repeat dosing in gene therapy applications.
In our preclinical studies of repeat microvesicle dosing in nonhuman primates, there was no appreciable toxicity or inflammation as measured by bloodwork including cytokine measurements, and no inflammatory changes seen on histopathologic review of tissues. In addition to their safety profile in single and repeat dosing, the large carrying capacity of these supra-100nm diameter microvesicles and their ability to simultaneously encapsulate multiple different editors within the same microvesicle drug product will enable their application to more complex diseases in which being able to correct multiple targets in a durable way will be critical for long-term cures.
This is an exceptional start that offers the prospect of the best dose repeatability available in the gene therapy delivery ecosystem today; and the opportunity to expand the landscape of diseases that can be treated.
About the Authors
Jonathan Thon, Ph.D., is the founder and CEO of STRM.BIO. Prior to founding STRM.BIO, he was CEO & CSO of Platelet BioGenesis. He has been a professor and lecturer at Harvard Medical School and is a frequent speaker at scientific conferences, entrepreneurship meetings, and thought leadership events. Jonathan earned his Ph.D. in biochemistry and molecular biology from the University of British Columbia and completed his postdoctoral research fellowship in hematology at Harvard Medical School.
Laura Goldberg, M.D., Ph.D. is the VP of Research and Development at STRM.BIO. Laura is an expert in hematopoietic stem cell biology and the role of extracellular vesicles in modulating cell function. Prior to joining STRM.BIO, Laura was an Assistant Professor of Medicine at Harvard University and hematologist in the Division of Hematology at Brigham and Women’s Hospital. She earned her M.D. and Ph.D. in molecular genetics and biochemistry from the University of Pittsburgh. She went on to complete her MD training with an Internal Medicine Residency at University of Pittsburgh then Hematology and Oncology Fellowship at Brown University.
- Muhuri M, et al. Durability of transgene expression after rAAV gene therapy. Molecular Therapy. 2022; 30(4): 1364-1380. DOI:https://doi.org/10.1016/j.ymthe.2022.03.004
- https://annualmeeting.asgct.org/abstracts/abstract-details?abstractId=14331
- https://annualmeeting.asgct.org/abstracts/abstract-details?abstractId=14378
- https://annualmeeting.asgct.org/abstracts/abstract-details?abstractId=13982
- Kim SK, Cho SW. The Evasion Mechanisms of Cancer Immunity and Drug Intervention in the Tumor Microenvironment. Front Pharmacol. 2022 May 24;13:868695. doi: 10.3389/fphar.2022.868695. PMID: 35685630; PMCID: PMC9171538.
- Fitzhugh CD, Cordes S, Taylor T, Coles W, Roskom K, Link M, Hsieh MM, Tisdale JF. At least 20% donor myeloid chimerism is necessary to reverse the sickle phenotype after allogeneic HSCT. Blood. 2017 blood-2017-03-772392
- Verdera HC, Kuranda K, Mingozzi F. AAV Vector Immunogenicity in Humans: A Long Journey to Successful Gene Transfer. Mol Ther. 2020 Mar 4;28(3):723-746. doi: 10.1016/j.ymthe.2019.12.010. Epub 2020 Jan 10. PMID: 31972133; PMCID: PMC7054726.
- Coughlan L. Factors Which Contribute to the Immunogenicity of Non-replicating Adenoviral Vectored Vaccines. Front. Immunol https://doi.org/10.3389/fimmu.2020.00909 (2020)
- Ertl HCJ. Immunogenicity and toxicity of AAV gene therapy. Front. Immunol. https://doi.org/10.3389/fimmu.2022.975803 (2022)
- Mohamed, M. et al. PEGylated liposomes: immunological responses. Sci Technol Adv Mater 20, 710-724, doi:10.1080/14686996.2019.1627174 (2019).
- Kenjo, E. et al. Low immunogenicity of LNP allows repeated administrations of CRISPR-Cas9 mRNA into skeletal muscle in mice. Nat Commun 12, 7101, doi:10.1038/s41467-021-26714-w (2021).
- Murphy, D. E. et al. Natural or Synthetic RNA Delivery: A Stoichiometric Comparison of Extracellular Vesicles and Synthetic Nanoparticles. Nano Lett 21, 1888-1895, doi:10.1021/acs.nanolett.1c00094 (2021).
- Bunggulawa, E. J. et al. Recent advancements in the use of exosomes as drug delivery systems. J Nanobiotechnology 16, 81, doi:10.1186/s12951-018-0403-9 (2018).
- Kao, C. Y. & Papoutsakis, E. T. Extracellular vesicles: exosomes, microparticles, their parts, and their targets to enable their biomanufacturing and clinical applications. Curr Opin Biotechnol 60, 89-98, doi:10.1016/j.copbio.2019.01.005 (2019).
- Wiklander, O. P. B., Brennan, M. A., Lotvall, J., Breakefield, X. O. & El Andaloussi, S. Advances in therapeutic applications of extracellular vesicles. Sci Transl Med 11, doi:10.1126/scitranslmed.aav8521 (2019).
- Kao, C. Y. & Papoutsakis, E. T. Engineering human megakaryocytic microparticles for targeted delivery of nucleic acids to hematopoietic stem and progenitor cells. Sci Adv 4, eaau6762, doi:10.1126/sciadv.aau6762 (2018).
- Driedonks T, Jiang L, Carlson B, Han Z, Liu G, Queen SE, Shirk EN, Gololobova O, Liao Z, Nyberg LH, Lima G. Pharmacokinetics and biodistribution of extracellular vesicles administered intravenously and intranasally to Macaca nemestrina. Journal of Extracellular Biology. 2022 Oct;1(10):e59.
- Saleh AF , Lázaro-Ibáñez E , Forsgard MA , Shatnyeva O , Osteikoetxea X , Karlsson F , Heath N , Ingelsten M , Rose J , Harris J , Mairesse M , Bates SM , Clausen M , Etal D , Leonard E , Fellows MD , Dekker N , Edmunds N . Extracellular vesicles induce minimal hepatotoxicity and immunogenicity. Nanoscale. 2019 Apr 4;11(14):6990-7001. doi: 10.1039/c8nr08720b. PMID: 30916672.
- Zhu X, Badawi M, Pomeroy S, Sutaria DS, Xie Z, Baek A, Jiang J, Elgamal OA, Mo X, Perle K, Chalmers J, Schmittgen TD, Phelps MA. Comprehensive toxicity and immunogenicity studies reveal minimal effects in mice following sustained dosing of extracellular vesicles derived from HEK293T cells. J Extracell Vesicles. 2017 Jun 6;6(1):1324730. doi: 10.1080/20013078.2017.1324730. PMID: 28717420; PMCID: PMC5505007
- G. Casella et al., Oligodendrocyte-derived extracellular vesicles as antigenspecific therapy for autoimmune neuroinflammation in mice, Sci. Transl. Med. 12 (568) (2020) eaba0599, https://doi.org/10.1126/scitranslmed.aba0599.
- Li X, La Salvia S, Liang Y, Adamiak M, Kohlbrenner E, Jeong D, Chepurko E, Ceholski D, Lopez-Gordo E, Yoon S, Mathiyalagan P. Extracellular Vesicle–Encapsulated Adeno-Associated Viruses for Therapeutic Gene Delivery to the Heart. Circulation. 2023 Aug 1;148(5):405-25.
- Gupta et al. Stem Cell Reviews and Reports (2021) 17:332–340.
- György B, Fitzpatrick Z, Crommentuijn MH, Mu D, Maguire CA. Naturally enveloped AAV vectors for shielding neutralizing antibodies and robust gene delivery in vivo. Biomaterials. 2014 Aug 1;35(26):7598-609.