What's In The Leukopak Matters For Cell Therapy Manufacturing
By Jennifer Chain, Ph.D., CABP, cellular starting material expert
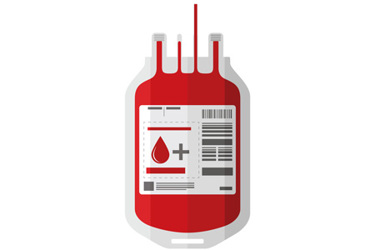
More than 500 clinical trials are currently underway exploring cellular therapies derived from healthy donor immune cells. While many factors influence the successful production and effectiveness of a cell therapy product, the quality of the cellular starting material is often overlooked and undervalued.
For allogeneic therapies that use unmodified or genetically modified lymphocytes or monocytes, non-mobilized leukopaks from healthy donors contain the right target cells to develop treatments for cancer, autoimmune diseases, infections, and other conditions. Without high-quality leukopaks from high-quality donors, the widespread success of advanced cellular therapies is not possible.
What Is A Leukopak?
Leukopaks are blood bags containing 100 mL to 300 mL of plasma, primarily filled with lymphocytes and monocytes (peripheral blood mononuclear cells, PBMCs). They also contain some contaminating platelets, red blood cells (RBCs), and granulocytes. For cell and gene therapy manufacturing, an ideal leukopak should have a hematocrit of ≤3%, contain over 90% PBMCs, and have minimal platelets. Typically, a leukopak containing 10 billion PBMCs is required to initiate most cell therapy manufacturing processes.
To achieve the desired concentration of PBMCs in a single bag, leukopaks are collected through automated leukapheresis. In this process, whole blood is drawn from a donor or patient, PBMCs are separated from the total white blood cell (WBC) fraction, and the remaining blood components are returned in a continuous loop.1 These “non-mobilized” leukopaks are collected from donors or patients who have not received any steroid or drug injections to mobilize cells from the bone marrow into the bloodstream prior to collection. This method is the most efficient for collecting lymphocytes and monocytes for use in cell-based applications such as adoptive and chimeric antigen receptor (CAR) therapies.
Why Do Leukopaks Matter?
The cell and gene therapy industry is currently dominated by autologous therapies, where a cancer patient’s cells are collected, processed into a therapy, and then reintroduced for treatment. Regardless of the quality of the starting material, the therapy is manufactured for the patient.
Manufacturing issues in autologous therapies lead to failures up to 25% of the time,2 causing those patients to miss out on their opportunity for treatment. The top reason cited for poor manufacturing outcomes is the quality of the starting material,2,3 including variations in cellular composition. Understandably, these variations are largely due to factors related to the patient’s disease state and previous treatments.2 However, it remains challenging to develop and implement a standardized manufacturing process that consistently produces an effective treatment for each patient when the patient-derived leukopaks are so variable.
For allogeneic cell therapy manufacture, the quality of starting material collected from healthy donors is also reported to be highly variable.4 Cell therapy developers frequently report inconsistencies in leukopaks from donor to donor and supplier to supplier. They observe significant variations in the number of PBMCs and the presence of contaminating RBCs, granulocytes, and platelets in each leukopak. Not only is consistency in healthy donor leukopak quality and makeup achievable, but as more allogeneic cell therapies advance to clinical trials, this consistency is paramount to successful manufacturing and treatment outcomes for patients.
Why Are Leukopaks So Variable?
Leukopak collection practices were initially developed for patients undergoing stem cell transplants and for healthy donors providing transplant material to type-matched patients. In these scenarios, it is crucial to maximize the number of cells collected.1 These collections could last up to eight hours per day over multiple days to gather sufficient cells for transplant. Early variations of this method were applied to healthy donors for research into allogeneic therapies, processing two or three total blood volumes (TBV) or a set number of liters of blood per donor when collecting a leukopak.1,4 These became typical collection practices, despite differences in donors’ TBVs, WBC counts, and target cell percentages, but these practices do not ensure the collection of 10 billion PBMCs.
Fortunately, it is now possible to better control the overall number of PBMCs in the leukopak by using an apheresis collection calculator that factors in these key donor variables. Without the use of a collection calculator, leukopaks may not have enough cells to start or complete the manufacturing process or may contain too many contaminating components that interfere with the isolation, activation, and function of therapeutic cells.
Comparing Yields With And Without Using A Collection Calculator
To illustrate the extent of leukopak variability when collected by targeting a set blood volume rather than a set cell count, refer to Table 1 below. The donors’ WBC count, percentage of target cells (% PBMCs), and TBV are hypothetical but representative of the variations among leukopak donors. When a donor’s collection is processed to two times TBVs (based on their height, weight, and gender), or if a fixed volume of 12 liters of blood is processed for every donor regardless of their size or cell counts, not all collections achieve the desired target of 10 billion cells. This results in wasted time and resources for cell therapy developers on leukopaks that don’t meet minimum requirements. However, by using each donor’s unique WBC count, %PBMCs, and TBV to estimate the process volume needed to collect 10 billion cells, all collections can meet the desired target.
Table 1: WBC yield and process volumes for hypothetical leukopak donors
Healthy Donor | WBC (cells/uL) |
%PBMC | TBV | Predicted PBMC if process: | Targeting 10B PBMC: | ||
2x TBV | 12L | Process Vol | Predicted PBMC | ||||
#1 | 8800 | 18% | 6.3L | 11.9B | 11.4B | 11.0L | 10.5B |
#2 | 5300 | 19% | 4.7L | 5.6B | 7.1B | 17.0L | 10.1B |
#3 | 6600 | 27% | 6.3L | 13.6B | 12.8B | 9.5L | 10.2B |
#4 | 6400 | 35% | 4.4L | 11.8B | 16.1B | 7.5L | 10.1B |
#5 | 5000 | 25% | 6.9L | 10.3B | 9.0B | 13.5L | 10.1B |
#6 | 4800 | 20% | 7.7L | 8.9B | 6.9B | 17.5L | 10.1B |
#7 | 5600 | 29% | 5.8L | 11.4B | 11.7B | 10.5L | 10.2B |
#8 | 7500 | 34% | 4.9L | 15.1B | 18.4B | 7.0L | 10.7B |
#9 | 9100 | 21% | 5.1L | 11.6B | 13.8B | 9.0L | 10.3B |
#10 | 3100 | 38% | 4.9L | 6.9B | 8.4B | 14.5L | 10.2B |
WBC = white blood cells, from complete blood count (CBC) report; PBMC = peripheral blood mononuclear cells, lymphocytes+monocytes, from CBC report; TBV = total blood volume; L = liters of blood; uL = microliters of blood; B = billion or x10e9 cells
Long Collection Windows Can Lead To Poor Quality
Collecting from a donor for too long can have significant consequences for both the donor and the leukopak. The longer the collection continues, the more likely donors are to experience the uncomfortable side effects from the anticoagulant used in leukapheresis,5 increasing the risk of collection failure and reducing their willingness to participate in future collections. Some collections targeting two times TBV or 12 L of blood yield more than 15 billion cells, as represented in the table above.
In these cases, overcollection is unpredictable and leads to waste in the manufacturing process. It may also shift industry demand toward higher-yield leukopaks, reducing the donor pool by excluding those with lower WBC or % PBMC counts or subjecting them to longer collections than they can tolerate. These practices affect the ability to meet supply needs, maintain a sufficient donor pool, recall ideal donors, and further contribute to non-standardized products. While it is impossible to collect exactly the target number of cells, working to ensure that leukopaks contain approximately the same number of cells each time helps to standardize downstream manufacturing processes.
Furthermore, the longer it takes to collect a leukopak from a donor, the more contaminating RBCs, granulocytes, and platelets are present in the final product. These contaminants negatively impact the quality of a leukopak for downstream manufacturing. For example, contact with RBCs suppresses T cell functions.6 When RBCs lyse during handling, shipping, or thawing of leukopaks, their cellular contents can affect the viability of neighboring PBMCs.6,7
Both viable and dying granulocytes in collected blood products compromise the integrity of T cells,8-10 affecting downstream testing, manufacturing, and cell function. The impact of granulocytes is amplified when leukopaks are cryopreserved. Granulocytes lyse upon thawing, releasing DNA and enzymes that damage surrounding PBMCs.11
While collecting platelets is somewhat unavoidable during leukapheresis, it is crucial to minimize their number in the bag. Platelet aggregation can cause issues with downstream cryopreservation, thawing, and isolation steps in manufacturing. Aggregated platelets bind to leukocytes, disrupting cell identification, isolation, and immune functions.12-15
Donors Matter
In the manufacture of allogeneic cell therapies, the selection of healthy donors is essential to the standardization of leukopak products, thereby reducing manufacturing variability. Many cell therapy companies have developed specific criteria to identify the best leukopak donors for their therapies, considering factors such as age, chronic health conditions, body mass index (BMI), and smoking history.
Some developers also qualify donors based on human leukocyte antigen (HLA) type, lifetime exposure to viruses, or latent viral reactivation. For therapies that rely on rare or specialized immune cell types like T stem cell memory, gamma delta, or NK cells, developers seek donors with higher numbers of these cells as well as better functionality in preclinical testing models. Regardless of the developer-specific criteria needed, donors must be qualified using FDA-compliant risk assessments and viral testing. Therefore, prequalifying and prescreening donors are necessary to ensure they meet all required and requested specifications. This ensures that the collected material is safe and suitable for the specific therapy being manufactured.
What Makes An Ideal Leukopak Donor?
Universal donor specifications that apply to all donors for all therapies are not feasible. However, ideal donor specifications can be defined for specific allogeneic therapies to maximize manufacturing success and therapeutic outcomes. Developers must determine these specifications for each of their therapies. Below are some key considerations when defining ideal donors:
Donor age
An individual’s immune system reportedly begins to decline functionally to protect from infections and cancer in the sixth decade of life.16 Nevertheless, patients receiving stem cell transplants from donors over 30 had lower overall survival rates than those receiving cells from donors under 30.17,18
In addition, patients receiving transplants from donors over 45 experienced higher graft failures than those receiving cells from younger donors.18 Although reports like these are limited, they have driven a preference in the industry for using leukopaks from the youngest donors possible. This phenomenon should be thoroughly tested before drastically restricting the donor pool for a specific therapy.
Donor race
Emerging evidence suggests a racial disparity in the effectiveness of autologous CAR T therapies, with Caucasian patients experiencing significantly better survival rates compared to Asian or African American patients.19,20 Various biological and socioeconomic factors could influence these results. For allogeneic therapies, it is currently unknown whether the donor’s race affects the treatment outcome in the patient, but it is an intriguing question that warrants investigation.
To date, leukopak donor pools are largely derived from blood and tissue donor databases, which contain a Caucasian majority.21 This raises the question of whether the therapeutic outcomes for non-Caucasian patients may be affected by this. Beyond HLA-type, should the donor and recipient be race-matched to increase the chance of treatment success?
Donor health history
Donors eligible for blood product collection used for direct transfusion must have key vital signs within range (e.g., temperature, blood pressure, heart rate) and avoid risk behaviors that could transmit relevant infectious agents. However, they are not restricted based on parameters like smoking habits, BMI, or chronic inflammatory conditions. For donors of leukopaks used in cell therapy manufacture, these factors may affect cellular functionality and therapy efficacy.
Donors who smoke have increased oxidative stress and inflammation, reportedly affecting therapeutic outcomes.22 Some donors with high BMI may have elevated inflammatory markers and altered metabolic profiles, impacting the quality and functionality of their T cells.23,24 However, donors with higher BMI are better able to tolerate the side effects of the leukapheresis anticoagulant and have a better chance of both completing a collection and returning for future collections. T cells from donors with chronic inflammatory conditions, such as autoimmunity, can exacerbate both immune rejection of the therapy and graft-vs-host disease in the recipient.25,26. It is essential to understand which health factors affect the manufacturability and potency of a specific therapy and ensure donors are prescreened to meet established requirements.
Immune cell population subtypes
To enhance manufacturing success for specific therapies, selecting donors with elevated levels of key immune cell populations can help. Upstream cell therapy manufacturing begins with isolating the key therapeutic population from the leukopak,27 whether it’s a type of T cell, NK cell, or another.
Donors with higher levels of the desired populations produce leukopaks that are more likely to succeed in manufacturing.2,3 A notable pitfall of targeting 10 billion PBMCs per collection is that donors have varied distributions of key lymphocyte and monocyte populations. For therapies involving rarer cell types, it is beneficial to work with leukopak suppliers to prequalify donors based on elevated levels of the specific cell type needed. This approach ensures that donors with lower levels of one key cell population but higher levels of another can be matched to different therapies, optimizing the limited donor pool.
Cellular performance in in vitro and in vivo therapy models
After screening for donors with elevated levels of target immune cell populations, the key to optimizing donor selection for leukopak collection and cell therapy success is evaluating the performance of donors’ cells in both in vitro culture and in vivo animal models.
Some cell therapy developers test donors’ cells for their ability to receive a CAR construct, expand, and kill tumor targets in cell culture and xenograft animal models. Then, only donors with the best-performing cells in these assays are recruited for leukopak collections to use in therapy manufacturing. This process helps developers, in collaboration with their suppliers, better understand donor factors that influence cellular performance and refine ideal donor specifications.
Conclusion
What’s in the bag of a leukopak can mean the difference between a successful and an unsuccessful manufacturing run for a cell therapy product. It could even mean the difference between an effective and ineffective treatment for the patient. As discussed here, both leukapheresis collection practices and donor selection practices influence the quality of the leukopak. As part of therapy development, it is important to study donor factors that contribute to successful manufacturing and potency of a specific therapy. Then, developers can present those essential parameters to leukopak suppliers capable of identifying ideal donors for that therapy program, collecting to a target cell count, and minimizing contaminants that affect key target cell function. These steps can ensure that best quality leukopaks go into the making of an effective cellular therapy.
References:
- https://pubmed.ncbi.nlm.nih.gov/29443409/
- https://ashpublications.org/bloodadvances/article/8/2/337/506509/Early-predictive-factors-of-failure-in-autologous
- https://www.ncbi.nlm.nih.gov/pmc/articles/PMC8462122/
- https://pubmed.ncbi.nlm.nih.gov/35085413/
- https://www.ncbi.nlm.nih.gov/pmc/articles/PMC10495341/
- https://www.ncbi.nlm.nih.gov/pmc/articles/PMC4344125/
- https://www.ncbi.nlm.nih.gov/pmc/articles/PMC3063218/
- https://pubmed.ncbi.nlm.nih.gov/16709924/
- https://pubmed.ncbi.nlm.nih.gov/19041316/
- https://www.ncbi.nlm.nih.gov/pmc/articles/PMC5573627/
- https://pubmed.ncbi.nlm.nih.gov/18220853/
- https://www.sciencedirect.com/science/article/pii/S0006497120716071
- https://www.mdpi.com/2079-7737/11/2/224
- https://www.ncbi.nlm.nih.gov/pmc/articles/PMC7382214/
- https://www.ncbi.nlm.nih.gov/pmc/articles/PMC6178087/
- https://doi.org/10.1513/annalsats.201602-095aw
- https://www.ncbi.nlm.nih.gov/pmc/articles/PMC7480347/#:~:text=Multivariable%20analyses%
20showed%20an%20association%20between%20donor%20age%20and%20OS, - https://www.ncbi.nlm.nih.gov/pmc/articles/PMC7948266/#:~:text=However%2C%20the%20
expanded%20use%20of%20matched%20unrelated%20donor,better%20outcomes%20than%20older
%20matched%20sibling%20donors.%2017 - https://ashpublications.org/bloodadvances/article/8/10/2589/516221/Race-and-insurance-real-world-insights-on-CAR-T
- https://ashpublications.org/blood/article/140/Supplement%201/623/491225/Racial-and-Ethnic-Differences-in-Clinical-Outcomes
- https://www.aabb.org/news-resources/news/article/2022/03/14/diverse-donor-pool-saves-lives
- https://bnrc.springeropen.com/articles/10.1186/s42269-024-01174-6
- https://www.ncbi.nlm.nih.gov/pmc/articles/PMC5507106/
- https://www.ncbi.nlm.nih.gov/pmc/articles/PMC3603502/
- https://www.ncbi.nlm.nih.gov/pmc/articles/PMC3262945/
- https://www.ncbi.nlm.nih.gov/pmc/articles/PMC3939032/
- https://www.ncbi.nlm.nih.gov/pmc/articles/PMC10791545/
About The Author:
Jennifer Chain, Ph.D., CABP, is a cellular therapy expert with 26 years of experience in T cell immunology, product development, blood banking, and consulting. She holds a Ph.D. in immunology and a Certified Advanced Biotherapies Professional credential from the Association for the Advancement of Blood and Biotherapies (AABB). She currently works as a consultant in the cellular starting material space, helping blood centers and cell therapy companies develop CSM collection and procurement programs and donor screening strategies. From 2016 to early 2024, she led efforts to collect leukopaks and bone marrow from over 1,000 healthy donors and developed novel blood- and cell-based culture materials for early-stage cell therapy companies. She volunteers and consults for AABB, where she engages in educational program development, strategic planning, and advocacy efforts in the field of cellular therapy. Reach her on LinkedIn or CSM Consulting’s website, www.cellsmatter.com.