The Pharmacological Treatment Pipeline For Traumatic Brain Injury
By Maddy Pliskin and Mavra Nasir, Back Bay Life Science Advisors
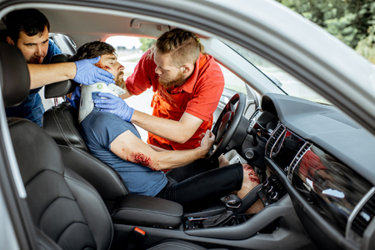
Traumatic brain injury (TBI) is defined as brain damage and dysfunction caused by an external physical force, often from sports injuries, car accidents, and falls. The global annual incidence for TBI is ~69 million, however, the majority of cases go undiagnosed.1 Current treatment for TBI depends on the extent of injury and severity of symptoms, with patients segmented as follows:
- Mild TBI, accounting for ~75 to 80% of all TBI cases, primarily requires rest and gradual resumption of typical activities.2 Overall treatment focuses on the management of post-concussive syndrome (PCS) through limiting visual and mental stimulation, avoiding light, and preventing further brain injuries.
- Severe and moderate TBI, accounting for ~20 to 25% of all TBI cases, require acute intervention to prevent and manage life-threatening secondary brain injuries within the hospital setting.2
- Patients with moderate TBI receive intravenous tranexamic acid (TXA) within the first 3 hours of injury, which is associated with reduced mortality. The antifibrinolytic agent TXA decreases post-traumatic coagulation, which can increase mortality tenfold if left untreated.3
- Patients with severe TBI require endotracheal intubation and may undergo treatment of hypotension and hypoxia using isotonic sodium chloride and ventilation, respectively. Furthermore, elevated intracranial pressure and cerebral perfusion may be treated surgically, depending on symptoms present.
Timely diagnosis of TBI remains a clinical challenge. The majority of TBI patients experience mild disease with delayed symptom onset, not seeking medical treatment unless their symptoms persist for a few weeks, or not seeking medical treatment at all. In contrast, moderate to severe TBI patients are more likely to seek immediate medical care due to more severe and time-sensitive injuries. Timely intervention for moderate to severe TBI is crucial to offset dangerous secondary events, and patients should be treated within 12 hours of injury to improve patient survival and outcomes.4
Approximately 43% of individuals who sustain moderate or severe TBI will experience long-term disabilities of cognitive, emotional, sensory, and motor functioning.5 Besides medical and functional consequences, impairments caused by TBI often affect professional and recreational involvement. A study by Ponsford and colleagues found that 10 years after injury, only 50% of the study population (n=141) resumed previous leisure activities, and fewer than 50% were employed after a decade.6 Furthermore, severe TBI has a 29.6% fatality rate, with a mean interval of 11.7 days between TBI and death.7
Despite TBI’s status as one of the leading causes of morbidity and mortality worldwide, no pharmacological interventions to mitigate the damaging sequelae are approved. Companies have attempted to develop pharmaceutical treatments for TBI; however, recent trials did meet statistical significance in their primary endpoint, while showing some benefit in subgroups:
- Vasopharm conducted a Phase 3 study of its small molecule ronopterin (VAS203), examining efficacy within 18 hours for patients with moderate to severe TBI. The trial did not meet the primary endpoint of improved extended Glasgow Outcome Score (eGOS) six months post-injury. However, in October 2021, Vasopharm announced that post-hoc analyses showed statistically significant differences between the placebo and treatment groups and the study’s data monitoring committee has given the drug a positive benefit-risk assessment, demonstrating ronopterin’s potentially clinical utility.
- Two Phase 3 studies investigating the efficacy of intravenously administered progesterone within 4 or 8 hours of injury did not meet primary endpoints (ProTECT [P.I. David Wright] and SyNAPSe [BHR Pharma], respectively). Despite preclinical animal studies supporting progesterone as an efficacious neuroprotective agent and promising Phase 2 studies with positive data readouts, Phase 3 studies did not significantly improve patient outcomes. For example, in the ProTECT trial (n=1195), the placebo group experienced greater eGOS improvement (50.5%) than the progesterone group (50.3%). Analyses of these studies cite various potential reasons for the surprising Phase 3 failures: animal studies inadequately recapitulating outcomes in TBI patients, improper characterization of TBI, and Phase 2 treatment dose and duration issues.8
There is significant unmet need for TBI therapies, leading to increased R&D efforts to discover new treatment approaches. We analyzed the clinical pipeline for new molecular entities at the proprietary level for TBI treatment to better understand the development landscape. There are 12 assets in clinical trials, with the majority of trials in early stages (Phase 1/2, n=9). Small molecules (n=4), cell therapies (n=3), and non-recombinant products (n=3) are the predominant modalities in the TBI pharmaceutical pipeline (Figure 1).
Figure 1. TBI Pharmaceutical Pipeline by Modality. TBI pharmaceutical products in clinical trials, broken out by trial phase and treatment modality
Small Molecules
There are four small molecules in development, including Vasopharm’s ronopterin in Phase 3. Three other small molecules are in Phase 2 clinical trials, including Oxeia Biopharmaceuticals’ OXE-103, Avanir Pharmaceuticals’ AVP-786, and NeuroVive’s NeuroSTAT (Table 1).
- OXE-103 is a first-in-class neurometabolic treatment for mild TBI, which freely crosses the blood-brain-barrier to help stabilize metabolic and energy brain dysfunction following a concussion. A Phase 2 clinical trial is being conducted to investigate the efficacy of OXE-103 in neurological healing and symptom management following mild TBI. Animal and laboratory studies found that OXE-103 restored energy metabolism, increased appetite, and reduced the effect of reactive oxygen species, which typically inundate the brain after injury.
- AVP-786 is being evaluated in a Phase 2 trial for the treatment of neurobehavioral disinhibition caused by TBI. AVP-786 is an NMDA receptor antagonist, sigma-1 receptor agonist, and CYP2D6 enzyme inhibitor that is approved for the treatment of pseudobulbar affect in ALS and MS.
Cell Therapies
After the primary injury that causes TBI, a plethora of secondary injuries may occur due to biochemical disruptions within the brain. Mesenchymal stem cells (MSC) have anti-inflammatory, immunosuppressive properties, and studies have shown that their infusion into an injured brain may prevent the occurrence or severity of secondary injuries while facilitating neuronal regrowth.9,10 Four early-stage cell therapy candidates include SanBio’s SB623 and Athersys’ MultiStem in Phase 2, Hope Biosciences’ HB-adMSCs in Phase 1/2, and American CryoStem’s ATCELL in Phase 1 (Table 1).
- SanBio’s SB623 showed safe and efficacious results for Phase 2 clinical trial in which moderate to severe TBI patients with chronic motor deficits received intracranial administration of MSCs. Six months after MSC implantation, participants receiving MSCs showed on average an 8.3-point improvement from baseline in motor skills, whereas the control group had an average improvement of 2.3 points from baseline. SanBio is preparing to file a Biologics License Application in Japan for SB623, as well as progress its global development program.
- Hope Biosciences’ autologous, adipose-derived MSCs (HB-adMSCs) are currently in a Phase 1/2 clinical trial to evaluate the safety and effects of HB-adMSC infusion on neurocognitive outcomes and neuroinflammation after TBI and hypoxic-ischemic encephalopathy. HB-adMSC is also in trial for various other neurological and rheumatological indications, as well as COVID-19.
Monoclonal Antibodies
Pinteon Therapeutics’ PNT001 is the only monoclonal antibody in clinical development for severe TBI. PNT001 is a tau antibody targeting a toxic neuroepitope cis-pT231 Tau, which phosphorylates and accumulates following TBI11 (Table 1). In June 2021, Pinteon secured $3 million from Advanced Technology International, a nonprofit contractor of the U.S. Department of Defense to run Phase 1 trials in TBI and also announced plans to initiate a Phase 1b study of PNT001 for patients with Alzheimer’s disease by the end of 2021. In addition, Pinteon extended a multiyear manufacturing collaboration with Lonza in July 2021, likely in preparation of the upcoming clinical studies.
- PNT001’s safety, tolerability, pharmacogenetics, and efficacy are currently being evaluated in a Phase 1 trial. A previous Phase 1 study of the safety of PNT001 demonstrated well-tolerability in healthy volunteers at doses that may lead to therapeutic drug concentration in the cerebrospinal fluid. While preclinical data from animal models of TBI have been promising and shown reduction in neurodegeneration, data in humans will be key in demonstrating clinical utility of PNT001 for patients with TBI.
Non-recombinant Products (Neuromuscular Blockers)
A common sequela of TBI is spasticity, the uncontrolled stiffening of muscles resulting from brain signal disruption, which usually presents within 12 months of injury onset. Spasticity caused by TBI often manifests within the upper or lower limbs and is especially dangerous for growing children as it can halt proper muscle and joint growth. Three non-recombinants in the TBI pharmaceutical pipeline include Merz’s NT 201 in Phase 3, Supernus Pharmaceuticals’ MYOBLOC in Phase 2/3, and Revance’s DAXI in Phase 2 (Table 1).
- The most advanced candidate in the TBI pharmaceutical pipeline, Merz’s NT 201, a botulinum toxin, is in Phase 3 trial for the treatment of lower limb spasticity caused by TBI. The study is currently enrolling and is expected to complete in Q4 2024.
- Supernus Pharmaceuticals’ MYOBLOC is currently in two Phase 2/3 clinical trials, one for adult upper limb spasticity due to TBI and one for adult lower limb spasticity due to TBI. Neither trial has data published yet, and both trials are estimated to complete in 2024.
- Revance Therapeutics’ daxibotulinumtoxinA (DAXI) is being evaluated in a Phase 2 clinical trial to treat adult upper limb spasticity due to TBI. Topline safety and efficacy data supports the advancement of DAXI into Phase 3 clinical trials, as the experimental group showed a statistically significant increase in muscle tone from baseline compared to the placebo group.
Recombinant Products
Only one recombinant product, Ipsen’s IPN10200, is in development for the treatment of upper limb spasticity caused by TBI. Similar to the aforementioned non-recombinant products, IPN10200 is a neuromuscular blocker to treat TBI-related limb spasticity (Table 1).
- Ipsen’s IPN10200 is a recombinant modified long-lasting neurotoxin comprised of botulinum toxin A’s light chain and botulinum toxin B’s heavy chain binding domain. The safety and initial efficacy signals of IPN10200 for the treatment of upper limb spasticity due to TBI is being assessed in a Phase 1 trial, estimated to recruit 209 patients.
Overall, the pipeline for TBI pharmaceutical treatments contains a wide variety of interventions and endpoint targets. Companies interested in developing pharmacological treatments for TBI can consider various developmental approaches to increase the treatment’s probability of success:
Target population:
- A pharmacological treatment for moderate to severe TBI would likely have high uptake due to life-threatening sequelae experienced by this group and unmet needs within the current SoC. There are currently no pharmacological interventions to treat TBI, and the dangerous biochemical disturbances following initial injury necessitates the development and commercialization of a new drug. Furthermore, a trial would be more likely to show a signal in this population and elevate a company’s profile if done successfully.
Time window of treatment:
- Treatments for moderate to severe TBI should retain high efficacy for up to 12 hours after onset of injury. Due to the speed and complexity of TBI pathophysiology, previously studied TBI drugs have exhibited diminished efficacy with increased time between injury and treatment. As more time passes, more secondary biochemical injuries occur and decrease functional outcomes.
Translational challenges with preclinical animal models:
- Despite promising preclinical animal studies, all treatments have failed to produce positive outcomes when translated to human TBI. Animal models inadequately represent the heterogeneity of human TBI; the pathobiology of TBI depends on injury severity and location, presence or absence of intracranial bleeding, co-morbidities, differences in gender, and various other factors. Furthermore, animal models use anesthesia, which may result in drug-drug interactions.
References
- Dewan, M. C. et al. Estimating the global incidence of traumatic brain injury. J. Neurosurg. (2019) doi:10.3171/2017.10.JNS17352.
- Bruns, J. & Hauser, W. A. The Epidemiology of Traumatic Brain Injury: A Review. Epilepsia (2003) doi:10.1046/j.1528-1157.44.s10.3.x.
- Zehtabchi, S., Abdel Baki, S. G., Falzon, L. & Nishijima, D. K. Tranexamic acid for traumatic brain injury: A systematic review and meta-analysis. Am. J. Emerg. Med. (2014) doi:10.1016/j.ajem.2014.09.023.
- Mohamadpour, M., Whitney, K. & Bergold, P. J. The importance of therapeutic time window in the treatment of traumatic brain injury. Front. Neurosci. (2019) doi:10.3389/fnins.2019.00007.
- Haarbauer-Krupa, J. et al. Epidemiology of Chronic Effects of Traumatic Brain Injury. J. Neurotrauma (2021) doi:10.1089/neu.2021.0062.
- Ponsford, J. L. et al. Longitudinal follow-up of patients with traumatic brain injury: Outcome at two, five, and ten years post-injury. J. Neurotrauma (2014) doi:10.1089/neu.2013.2997.
- Song, S. Y. et al. Analysis of mortality and epidemiology in 2617 cases of traumatic brain injury: Korean Neuro-Trauma Data Bank System 2010-2014. J. Korean Neurosurg. Soc. (2016) doi:10.3340/jkns.2016.59.5.485.
- Stein, D. G. Embracing failure: What the Phase III progesterone studies can teach about TBI clinical trials. Brain Injury (2015) doi:10.3109/02699052.2015.1065344.
- Stocchetti, N. et al. Neuroprotection in acute brain injury: An up-to-date review. Critical Care (2015) doi:10.1186/s13054-015-0887-8.
- Hasan, A. et al. Mesenchymal stem cells in the treatment of traumatic brain injury. Frontiers in Neurology (2017) doi:10.3389/fneur.2017.00028.
- Albayram, O. et al. Cis P-tau is induced in clinical and preclinical brain injury and contributes to post-injury sequelae. Nat. Commun. (2017) doi:10.1038/s41467-017-01068-4
About the Authors:
Maddy Pliskin joined Back Bay Life Science Advisors as part of its Summer Analyst Program, working on market entry and product valuation projects. She is currently studying neuroscience, biology, and public health at Brandeis University, where she is involved in academic and clinical research. Pliskin is writing her senior honors thesis with the Valera Lab at Harvard Medical School. Contact her on LinkedIn.
Mavra Nasir, Ph.D., is a senior consultant at Back Bay Life Science Advisors, where she supports strategic engagements across a range of therapeutic areas including rare diseases, hematology, oncology, and metabolic diseases for biopharma and medtech companies and their investors. She joined Back Bay after receiving her Ph.D. in quantitative biomedical sciences from Dartmouth College. She has written on the complexities of life sciences development, including the NASH treatment pipeline and the protein degradation clinical and investment landscape. Connect with her at www.bblsa.com.