The ABCs of Oncology: Looking Beyond Alpha-Beta Cells for Cell Therapies Part 1
By Kyle O’Neil and Peter Bak, PhD, Back Bay Life Science Advisors
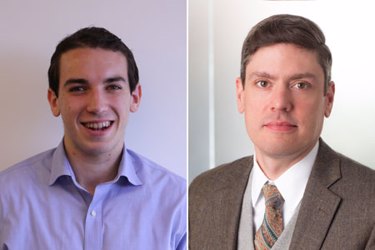
Cell therapies for cancer, particularly chimeric antigen receptor (CAR)-modified αβ T cells, have rapidly proven their utility. However, as limitations of these therapies have become clearer, interest in alternative approaches has grown. A second generation of companies built on several scientific discoveries is advancing cell therapies of the innate immune system (such as γδ T and NK cells) to address the limitations of CAR T-cell therapies. With established investor interest, the potential of these approaches to impact the standard of care has become clear. The translatability of next-generation cell therapies will rely on developing a strategy to achieve proof-of-concept and optimal clinical positioning, which may lead to improved patient outcomes, broader interest in the biopharmaceutical community, and returns for investors.
In this article, part one of a two-part series, we explore the evolution of cell therapies of the innate immune system (such as γδ T and NK cells) to understand why these approaches offer great hope for oncology and the potential value they may offer second-generation cell therapy companies. Next week we will dive deeper into this burgeoning industry and the potential commercial impact these therapies may offer.
Over the last decade, scientific advances in understanding the immune system have transformed cancer care. Improved knowledge of the adaptive immune response, driven by T and B cells, has allowed scientists and clinicians to harness the tumor-killing power of the immune system. Cell therapies, or “living drugs,” are among the most effective new medicines, in which αβ T cells are removed from the body and modified before being returned to the patient.
Currently, the most efficacious oncology cell therapies use T cells modified by a chimeric antigen receptor (CAR), which have demonstrated groundbreaking success in hematologic malignancies. However, CAR T-cell therapies are still constrained by toxicities, manufacturing logistics, and in the case of solid tumors, the ability to both travel to the site of tumor growth and overcome the immunosuppressive effects of the local tumor microenvironment. Although several approaches in early development are aiming to address these limitations by further modifying CAR T-cell therapies, using alternative immune cells may be a more effective strategy.
Cell therapies using the cells of the innate immune system may address crucial gaps in patient care. Natural killer (NK) and gamma-delta (γδ) T cells—both components of the innate, rather than the adaptive, immune system—can utilize a variety of properties for tumor killing and may hold the key to commercial success. This article examines the scientific rationale for NK and γδ T-cell therapies and assesses both the investment landscape in and company development around next-generation cell therapies in oncology.
Evolution of CAR T-Cell Therapies
T cells are characterized by their distinct T-cell receptor (TCR), which in approximately 95% of all T cells, consists of two glycoproteins: the α (alpha) and β (beta) chains. αβ T cells have a variety of roles in the adaptive immune response that make them attractive, but imperfect, tumor cell killers. As αβ T cells mature, they are selected to recognize small portions of intracellular proteins—nine to ten amino acid sequences—that are presented on the cell surface bound to major histocompatibility complex (MHC) proteins. When a unique αβ T cell receptor recognizes a distinct peptide-MHC protein, the αβ T cell becomes activated, begins proliferating, and differentiates into an αβ T cell subset, which can directly kill the cell. This mechanism has evolved to allow the body to recognize and destroy cells infected with foreign material—viruses, intracellular pathogens, and interestingly, the mutated proteins that arise as a result of tumor growth. However, cancerous cells that successfully proliferate in some cases have evolved mechanisms to “cloak” themselves from a T-cell response. Therefore, researchers have long been interested in harnessing the killing ability of αβ T cells and recently uncovered a groundbreaking way to help them recognize tumors previously seen as healthy tissue: the CAR T cell.
CAR T-cell therapies are the result of αβ T cells that are genetically engineered to express chimeric antigen receptors (or CARs) targeting tumor associated antigens (TAAs), such as CD19. Although αβ T cells have an intrinsic capacity to target TAAs, CAR T-cells cells are armed with an antibody to increase their killing capacity when they recognize a TAA. More specifically, CAR T-cells cells are engineered to express several components, including an antigen-binding domain, a hinge, a transmembrane domain, and an intracellular signaling domain. Ultimately, this enables the CAR T-cells cell to recognize native proteins, removing the restriction of peptide-MHC recognition. When the antigen-binding domain recognizes the TAA, the CAR T-cells cells become constitutively active, secreting cytokines, cloning, and activating killing mechanisms in a reaction that would not occur without the engineered binding domain.
Despite clinical success, this approach has several biological limitations. First, the CAR T-cells cells are extremely persistent, leading to actively armed and proliferating cells that can linger long after a tumor is eradicated, causing severe side effects. Second, antigen escape has been documented, with cancerous cells losing or downregulating the CAR T-cells TAA target. Lastly, CAR T-cells only recognize TAAs on the cell surface, limiting the available targets to 1% of all cellular proteins. Each biological limitation of CAR T-cells is linked to a major challenge. Cytokine release syndrome is a side effect of an overactive immune response occurring in more than 75% of patients, antigen escape has been documented in 30% of patients, and limiting targets to the cell surface restricts the patients eligible to receive CAR T-cells (1, 2). Therefore, differentiated strategies to improve cell therapies are of both scientific and clinical interest.
In contrast to αβ T cells, both NK and γδ T cells play roles in the innate immune system. While the role of NK cells in providing rapid responses to invading pathogens is well documented, γδ T cells are not as well understood, and they account for only ~5% of T cells in the body. There are several biological advantages for using NK and γδ T cells in cell therapies. NK and γδ T cells do not require MHC proteins, meaning they do not require prior antigen exposure to begin to act on tumor cells directly.
In the case of CAR-NK and CAR-γδ T-cell therapies, this limits TAA escape, as CAR-NK and CAR-γδ T-cell therapies retain an intrinsic capacity to recognize tumor cells through receptors native to NK and γδ T cells. More specifically, NK cells express either activating or inhibitory germline- encoded receptors, which have killing mechanisms complementary to T cells that limit TAA escape.
This feature means the cells do not recognize the many “marker-of-self” proteins produced by the body, enabling the cells harvested from one patient to be used in other patients without causing graft-versus-host disease and making an “off-the-shelf” approach viable for CAR-NK and CAR- γδ T-cell therapies. NK and γδ T cells have limited clonal expansion and persistence capabilities in the body, ultimately restricting the occurrence of side effects.
Research into NK and γδ T cells has shown both cell types have an abundant cytokine secretion capacity, activating the adaptive immune response. By indirectly activating the adaptive immune response, NK and γδ T cells stimulate the anti-tumor response without over-activating killer cells that can cause toxicities.
In part two, we will explore the future of these cell therapies and their potential commercial implications.
About the Authors
Kyle M. O’Neil is an Analyst at Back Bay Life Science Advisors, where he provides strategic guidance to biotech, pharmaceutical, and medical device developers across an array of therapeutic areas.
Peter Bak, Ph.D., a Senior Vice President at Back Bay Life Science Advisors, has more than ten years of experience with a broad range of research approaches—cellular, molecular and biochemical—and fields—from immunology and infection, with emphasis on oncology. Contact the authors at info@bblsa.com.