Surmounting The Challenges To Realizing The Potential Of CGT For Neurological Disorders
By Booma Yandava, M.S., MBA, Principal at Alliance Partners
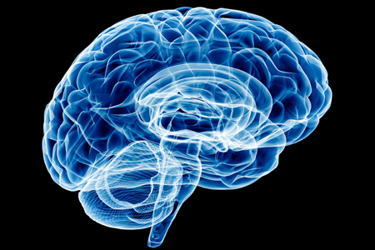
In the United States alone, incidences of rare diseases have surged, now numbering over 6,800 and affecting between 25 and 30 million people. This surge is paralleled by a burgeoning array of therapeutic solutions that bring significant relief to patients grappling with a wide spectrum of monogenic disorders, including those of a neurological nature. Recent data from an IQVIA report highlights a 31% growth in the central nervous system (CNS) pipeline over the past five years, underscoring the profound medical need in the field of neurological diseases. Analysis of clinical trials from 2022 to 2023 reveals a focused effort to address neurological disorders, driven by advancements in molecular understanding, enhanced molecular diagnostics, the integration of molecular insights with clinical outcomes, and refined clinical trial designs. It is evident that cell and gene therapy CGT for neurological disorders is experiencing a renaissance.
Figure 1: Data source: IQVIA Report- 2022, FDA website, published reports
Challenges and Innovations
The CNS is composed of highly specialized cell types with subpopulations associated with the pathogenesis of many neurological disorders. Despite great strides in understanding pathophysiology and in targeted gene delivery for restoring normal functionalities, neurological disorders present a complex, multifactorial challenge, characterized by diverse disease patterns, often with varied severity and resistance to conventional therapies. Unlike other systems, the CNS is protected by the blood-brain barrier, which prevents any toxic substances from entering the brain but at the same time poses challenges to route of administration of targeted therapies.
CGT stands out by offering long-term, targeted corrections to disease-specific etiologies, promising significant benefits for a range of debilitating neurological conditions. Therapeutic strategies vary, from plasmid-based expression systems to vector-based gene delivery aimed at replacing mutant genes to the modulation of gene expression through non-coding RNA, silencing the genes responsible for specific disease phenotypes. RNA-based therapies are at the forefront, while gene-editing techniques, notably those using CRISPR/Cas9, are showing potential (1, 2). An assessment of ongoing clinical development programs indicates that predominant clinical trials utilize adeno-associated virus (AAV)-based delivery systems in the neurological disease area (figure 2)
Figure 2: Data source: https://clinicaltrials.gov, and published reports
AAV-based therapies, favored for their broad cell-targeting abilities, are undergoing significant improvements to enhance specificity, reduce immunogenicity, and cross the blood-brain barrier effectively through extensive sequence analysis. Notable improvements in AAV-based gene delivery are novel capsids and tissue-specific promoters. Strain-improved AAVs offer the ability to infect a wide range of dividing cells such as astrocytes and non-dividing cells such as neurons. Tremendous efforts are being invested in developing cell type specific versions of AAV (3) - for example, AAV1, AAV5, AAV8, and AAV9 transduce neurons, astrocytes, and oligodendrocytes, while AAV2 transduces primarily neurons. Key desirable factors are a vector’s ability to target a broad range of cell types, low immunogenicity, and crossing of the blood-brain barrier. Though AAV vectors are immunogenic, improvements in serotypes have tried to address this challenge effectively by various codon optimization strategies. For example, AAVrh10, discovered more recently, also mediates effective CNS transduction after intravenous injection into mice. Several studies have shown that intravenous administration of AAVrh10 achieved similar or higher transduction efficiency than AAV9 in all brain regions studied in a mouse model.
Table 1: Transgene capacity of various delivery systems
In addition to AAV, lentivirus-mediated gene delivery is also under clinical development for cerebral adrenoleukodystrophy (CALD), Parkinson’s disease, and refractory focal epilepsy. These vectors offer the advantage of packaging up to 10 kb of single-stranded RNA (ssRNA). Other viral delivery systems utilized are based on herpesvirus and retrovirus. Due to the possibility of their integration into the host genome leading to insertional mutagenesis, these vectors are less widely used than AAV. Moreover, the prohibitive cost of manufacturing is a major concern, along with the loss of expression in some instances.
Non-viral delivery systems based on cationic lipid polymers and nanoparticles have been gaining prominence, especially with RNA-based modalities such as anti-sense RNA, circular RNA, and other variations, along with naked DNA molecules. Although these are significantly less expensive to manufacture in larger quantities, often the expression levels and tissue specificity are not comparable with that of viral vectors. Another non-viral delivery system is making waves in the field: the revolutionary CRISPR-Cas9 technology, which offers new avenues for treating a range of neurological conditions. Non-viral vectors have been found promising for the targeted delivery of CRISPR/Cas9, due to cost effectiveness and relative ease of delivery. With the advent of the CRISPR-Cas9 gene-editing technology, disease-causing mutations can now be targeted and edited in neural cells, potentially offering therapeutic solutions for a wide range of neurological disorders. This approach is currently being evaluated in clinics for refractory viral keratitis and Leber congenital amaurosis inherited blindness. RNA-based therapies, including small interfering RNAs (siRNAs) and antisense oligonucleotides (ASOs), have shown promise in treating neurological disorders by targeting specific RNA molecules associated with disease pathology.
Advances in stem cell biology have led to the generation of induced pluripotent stem cells (iPSCs) from patients' own cells. These iPSCs can differentiate into specific neuronal cell types, allowing for disease modeling and drug screening, as well as potential sources of transplantable cells. Cellular therapy derived from autologous bone marrow–derived mesenchymal stem cells (MSCs), induced to secrete elevated levels of neurotrophic factors (NTFs) are under phase-3 clinical trial for ALS and phase 2 for progressive multiple sclerosis (MS) and Alzheimer’s disease. Retinoid progenitor cells (RPC), derived from retinal cells, are also under clinical trials. While RPC and iPSC may not elicit immune response and tissue rejection, they may pose a risk of tumorigenic potential due to their undifferentiated nature.
A therapeutic avenue that is less explored is the conversion of glial cells into functional neurons for replenishing neuronal loss associated with neurodegenerative diseases and brain injury. Although the expression of transcription factors was explored to modulate cellular differentiation paths in the CNS, these studies were not successful and not easily translatable to therapeutic use. In a recent study by Zhou et.al, it was demonstrated that downregulation of a single RNA-binding protein, polypyrimidine tract-binding protein 1 (Ptbp1), using in vivo viral delivery of a CRISPR system CasRx, resulted in the conversion of glia into retinal ganglion cells (RGCs) leading to the alleviation of disease symptoms associated with RGC loss, offering a promising treatment option for disorders due to neuronal loss (7).
Realizing the Potential – Tools and Clinical Development
Clinical development in neurological disorders would be challenging without the immense progress in understanding disease etiology and disease patterns, early detection, and molecular diagnosis. Research in these areas includes three-dimensional (3D) modeling for understanding disease progression, therapeutic interventions, tools such as optogenetics and chemo-genetics that allow for manipulating neuronal activity with high precision for end-point designs, and advances in electrode design and targeting methods that have improved the effectiveness and safety of therapeutic approach for movement disorders. A combination of high-throughput genome analysis, functional studies, biomarkers, and clinical validation in undiagnosed patients has led to discoveries of disease-causing genes and the translation of results into improved diagnosis, treatment, and drug trials in rare neurological disorders. For example, although the disease-causing expansion of a CAG repeat was identified in 1993 as an etiological agent for Huntington’s disease, further studies of families affected by Huntington’s disease in combination with advances in molecular biology has led to the development of several new therapies based on RNA interference, antisense oligonucleotides, and small molecules that disrupt gene transcription, and some of these are in clinical trials. In the field of neuro-oncology, diagnostic tools such as immunohistochemical prediction of 1p/19q loss in diffuse glioma and molecular profiling for CNS lymphoma have led to better stratification and molecular diagnosis of meningioma and glioblastoma. Notable progress has also been in enhancing our understanding of the molecular mechanisms underlying neurological disorders. For instance, research has revealed a correlation between the development of Alzheimer's disease and the expression of calcitonin gene-related peptide (CGRP), which is found in various dorsal ganglion cells and is implicated in spinal cord injuries (6). This increased molecular insight is a critical step forward in understanding not only Alzheimer’s disease but also other disorders where the central nervous system is affected. Several pre-clinical studies have shown the use of iPSCs for disease modeling, drug screening, and testing cell‑based therapy for Alzheimer’s disease under in vitro conditions. This offers a disease-in-a-dish model that can be applied to other disorders.
The identification of reliable biomarkers (for example, β-amyloid, total tau, and phosphorylated tau in Alzheimer’s disease), or neuroimaging data has helped in early disease detection, in tracking disease progression, and in assessing treatment efficacy. The development of genetically modified animal models that mimic human neurological diseases has been instrumental in preclinical testing of therapeutic interventions. However, although rodent models have been instrumental in gaining an understanding of the pathogenesis of neurological diseases, they do not exhibit neuronal loss. On the other hand, the use of non-human primates is prohibitive in terms of cost and their long-life cycle. Therefore, other animal models, such as pigs with knockout genes or specific transgene expressions, have proven informative (4). Analyzing large datasets using machine learning and artificial intelligence algorithms can identify novel therapeutic targets, predict disease outcomes, and optimize treatment strategies. For example, Asif. M et.al (2020), by using machine learning, demonstrated that milder and more severe clinical presentations of autism spectrum disorder (ASD) can have distinct underlying biological mechanisms (5). In addition, the establishment of regulatory pathways and guidelines specific to cell and gene therapies has facilitated the translation of promising discoveries into clinical trials, accelerating the development of treatments for neurological diseases.
Significant advancements are being made in the realm of gene therapy for neurological disorders, thanks to various discoveries, tools, and techniques. Notable progress includes the development of delivery systems capable of crossing the blood-brain barrier (BBB), enhanced transgene expression that reduces dosage requirements, and the overcoming of substantial manufacturing challenges. Furthermore, the application of machine learning is revolutionizing our approach to these complex diseases. By leveraging these advanced computational techniques, we can deepen our understanding of disease mechanisms, which in turn facilitates early diagnosis, disease progression, and stage-specific treatment. This technology also plays a pivotal role in the better design of therapeutic avenues, offering more targeted and effective treatments. Cell and gene therapy for neurological diseases is here to stay.
However, the journey ahead remains challenging. Currently, only 25–30% of neurological diseases are detectable, let alone treatable, highlighting a significant gap, and underscoring the need for broader diagnosis and therapeutic avenues. Incorporating innovative technologies, such as CRISPR/Cas9, and understanding the molecular basis of neurological disorders are crucial in shaping the future of gene therapy. These approaches, combined with a more holistic, multidisciplinary strategy and a strong emphasis on understanding the patient’s journey, will lead to more accurate diagnoses and more effective therapies for neurological conditions.
Bibliography
- Davidson, Beverly L., and Ryan L. Boudreau. "RNA interference: a tool for querying nervous system function and an emerging therapy." Neuron 53.6 (2007): 781-788
- Jagannath, Aarti, and Matthew Wood. "RNA interference based gene therapy for neurological disease." Briefings in Functional Genomics and Proteomics 6.1 (2007): 40-49.
- Ingusci, Selene, et al. "Gene therapy tools for brain diseases." Frontiers in Pharmacology 10 (2019): 724.
- Yin, Peng, et al. "New pathogenic insights from large animal models of neurodegenerative diseases." Protein & Cell 13.10 (2022): 707-720
- Asif, Muhammad, et al. "Identification of biological mechanisms underlying a multidimensional ASD phenotype using machine learning." Translational psychiatry 10.1 (2020): 43.
- Singh, Yogendra, et al. "Calcitonin gene‐related peptide (CGRP): A novel target for Alzheimer's disease." CNS neuroscience & therapeutics 23.6 (2017): 457-461
- Zhou, Haibo, et al. "Glia-to-neuron conversion by CRISPR-CasRx alleviates symptoms of neurological disease in mice." Cell 181.3 (2020): 590-603.
Booma Yandava, Principal, Alliance Partners, Wellesley, MA
Booma Yandava provides consultative services to transactions and licensing in support of early-stage discovery programs leading to commercialization. For the past 15 years, she has held leadership roles across business development, transactions, R&D strategies, and C-suite support in the biopharma industry. Along with her wider therapeutic expertise, she is frequently asked to contribute to scientific thought leadership. She has enthusiastic interest in cell and gene therapy, CNS, and rare diseases. Booma holds a master’s in business administration from Babson’s Olin School of Management and a master’s degree in genetics.