iPSC-based Gene Therapy: Applications And Challenges
By Jack (Jie) Huang MD, Ph.D., chief scientist, founder/CEO, CSTEAM biotechnology
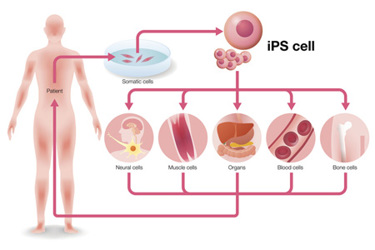
Since the discovery by Shinya Yamanaka in 2006, induced pluripotent stem cells (iPSCs) have revolutionized the fields of regenerative medicine and gene therapy.1 iPSCs are generated by reprogramming adult somatic cells, such as skin or blood cells, into a pluripotent state, meaning they can develop into any cell type in the human body. This is achieved by introducing four key transcription factors: Oct3/4, Sox2, Klf4, and c-Myc.2 These factors reprogram adult cells into a stem cell-like state similar to embryonic stem cells (ESCs), but without the ethical and legal issues associated with ESCs.
The potential of iPSCs is enormous. They provide a flexible and scalable platform for disease modeling, allowing scientists to replicate disease processes in vitro and test therapeutic interventions.3 This is particularly valuable for understanding genetic diseases at the cellular level. In addition, iPSCs have become an important tool for drug discovery, making it possible to test new drugs in a disease-specific cellular environment, improving drug safety and accelerating the development of personalized treatments.
In the field of gene therapy, iPSCs offer a unique opportunity to repair genetic defects. Patient-specific, genetically corrected cells can be created for autologous transplantation by deriving iPSCs from a patient's own cells, correcting genetic mutations using gene editing tools such as CRISPR/Cas9, and then differentiating these corrected iPSCs into desired cell types.4 This personalized approach eliminates the risk of immune rejection, a common complication of traditional transplantation therapies, and offers lifelong cures for genetic diseases such as sickle cell anemia, Duchenne muscular dystrophy, and cystic fibrosis.5 In addition, iPSCs hold great promise for regenerative medicine. They can be used to generate healthy tissue for transplantation, such as heart cells for repairing damaged heart tissue, neurons for treating neurodegenerative diseases, and pancreatic beta cells for treating diabetes.6 The ability to generate a patient's own healthy tissue opens new avenues for treating a wide range of diseases, including those for which there are currently no effective therapies.
This review discusses the current progress, applications, challenges, and future prospects of iPSC-based gene therapy, focusing on its potential in treating genetic diseases, cancer, and age-related disorders.
Figure 1: Induced pluripotent stem cell (iPSC) technology is being used to create immune cells, such as T cells and natural killer (NK) cells, which are genetically engineered to recognize and eliminate cancer cells.
Applications Of iPSC-Based Gene Therapy
Genetic Disorder Treatment
Gene therapy based on iPSCs offers great potential for treating a variety of genetic diseases, such as sickle cell anemia, cystic fibrosis, and Duchenne muscular dystrophy.7 This innovative approach enables scientists to create patient-specific iPSCs that are reprogrammed from the patient's own somatic cells. These iPSCs can be genetically corrected in vitro to repair disease-causing mutations and then differentiated into the desired cell type for transplantation, providing personalized and targeted treatment options with minimal risk of immune rejection.
One of the most promising applications of iPSC-based gene therapy is the treatment of sickle cell anemia, an inherited blood disorder caused by mutations in the hemoglobin gene, which results in the production of abnormal red blood cells.8 These sickle cells tend to aggregate and block blood flow, causing pain, organ damage, and other serious complications. In this case, patient-derived iPSCs can be genetically modified using tools such as CRISPR/Cas9 to repair the mutated hemoglobin (HBB) gene. The corrected iPSCs can then be differentiated into hematopoietic stem cells (HSCs), which are precursors to red blood cells. Once transplanted back into the patient, these corrected HSCs produce healthy, functioning red blood cells, effectively curing the disease. Preclinical studies have shown this approach to be successful, with iPSC-based gene correction restoring normal blood function and alleviating disease symptoms in animal models, offering hope for eventual clinical application.
Similarly, iPSCs have been used to model and test therapeutic interventions for cystic fibrosis, a genetic disease caused by mutations in the CFTR gene.9 This gene encodes a protein responsible for regulating the flow of salt and fluid in and out of cells, and mutations result in thick mucus that causes severe respiratory and digestive problems. In iPSC-based models of cystic fibrosis, patient-derived cells can be reprogrammed into iPSCs, and then the defective CFTR gene is genetically corrected. These corrected cells can be differentiated into relevant cell types, such as lung epithelial cells, for transplantation. By correcting the genetic mutations that cause cystic fibrosis, these iPSCs have the potential to restore normal cellular function, providing a breakthrough approach to treating this life-threatening disease. Additionally, these iPSC-based models provide a platform for testing new drugs and treatments, accelerating drug development efforts for cystic fibrosis.
iPSC-based gene therapy also has made significant progress in treating Duchenne muscular dystrophy (DMD), a devastating genetic disease characterized by progressive muscle degeneration.10 DMD is caused by mutations in the dystrophin gene, resulting in a loss of dystrophin, a protein required to maintain muscle integrity. Patient-derived iPSCs can be used to correct dystrophin gene mutations, and these corrected cells can differentiate into muscle cells. Once transplanted back into the patient, these cells have the potential to repair damaged muscle tissue, slow disease progression, and improve muscle function. Early preclinical studies have shown promising results, raising hopes for future therapeutic applications in humans.
iPSC-Based Cancer Therapy
iPSC-based cancer treatment is a rapidly evolving area of research that offers the potential for personalized and highly targeted immunotherapies.11 iPSC technology is being used to create immune cells, such as T cells and natural killer (NK) cells, that are genetically engineered to recognize and eliminate cancer cells. These immune cells can be derived from iPSCs and engineered to express chimeric antigen receptors (CARs) or T cell receptors (TCRs) that specifically target cancer antigens.
One of the most important applications of iPSC technology is the development of CAR T cell therapy, which has shown remarkable success in treating blood cancers such as leukemia and lymphoma.12 CAR T cells are engineered to express receptors that recognize specific proteins on the surface of cancer cells, thereby triggering an immune response that destroys the tumor. However, the application of CAR T cell therapy in solid tumors has proven challenging due to the immunosuppressive tumor microenvironment (TME) that impedes effective T cell infiltration and activity. iPSC-derived CAR T cells offer a renewable and scalable source of immune cells that can overcome these obstacles by engineering immune cells to resist immunosuppressive signals or enhancing their ability to infiltrate solid tumors.
In addition to CAR T cells, the potential of iPSCs to generate dendritic cells (DCs) for cancer vaccines is also being explored.13 Dendritic cells are professional antigen-presenting cells (APCs) that play a crucial role in stimulating T cells to recognize and attack tumor cells. iPSCs can be differentiated into dendritic cells loaded with tumor-specific antigens and, once introduced into the patient, these cells activate the immune system to initiate a specific anti-tumor response. This approach improves the effectiveness of immunotherapy, especially for patients whose immune systems cannot effectively recognize cancer cells. Therefore, iPSC-derived cancer vaccines can become an important component of personalized cancer treatment, helping to train the immune system to fight cancer more effectively.
Age-Related Diseases and Regenerative Medicine
iPSC-based therapies also hold promise for treating age-related diseases and regenerating damaged tissue. With age, stem cell function declines, leading to a loss of tissue repair capacity. iPSC technology offers a way to rejuvenate tissues by generating healthy young cells to replace damaged or aged cells.14 For example, iPSCs have been used in preclinical studies to generate dopaminergic neurons for Parkinson's disease, where the loss of dopamine-producing neurons leads to motor dysfunction. By transplanting iPSC-derived neurons, researchers restored dopamine levels and improved motor function in animal models, suggesting that this approach could be a viable therapy for Parkinson's disease in the future. In the context of macular degeneration, a leading cause of blindness in the elderly, iPSC-derived retinal cells have been used to replace damaged retinal pigment epithelial (RPE) cells in patients. Early clinical trials have shown promising results, with patients experiencing stabilization or improvement in vision after receiving iPSC-based therapies.
Challenges To Overcome In iPSC-Based Gene Therapy
Safety Concerns
One of the main concerns with iPSC-based therapies is the risk of tumor formation.15 During the reprogramming process, cells may undergo mutations that can lead to oncogenic transformation. In addition, transcription factors used for reprogramming, such as c-Myc, are known to be involved in tumorigenesis. Ensuring the genetic stability of iPSCs and their derivatives is essential for their safe use in clinical applications. To mitigate these risks, alternative reprogramming methods that do not rely on potential oncogenic factors have been developed, including small molecule-based reprogramming and the use of non-integrating viral vectors. These methods help reduce the likelihood of introducing mutations during the reprogramming process and improve the safety of iPSC-based therapies.
Immune Rejection
Although iPSCs are often referred to as "patient-specific," recent studies have shown that iPSCs can still trigger an immune response when transplanted back into patients.16 The reasons behind this immune rejection are not fully understood but may be related to epigenetic modifications or the expression of immunogenic proteins during the reprogramming process. To address this issue, researchers are exploring ways to make iPSC-derived cells more compatible with the patient's immune system. This includes gene editing to eliminate immunogenic markers or using universal donor iPSCs that are engineered to avoid immune detection.
Efficiency of Differentiation and Integration
Another challenge lies in efficiently differentiating iPSCs into the desired cell types and ensuring that they properly integrate into the patient's tissues.17 Differentiation protocols for generating specific cell types, such as neurons, hepatocytes, or pancreatic beta cells, are still being optimized. In addition, cells must functionally integrate into existing tissues to have a therapeutic effect. For example, in the case of type 1 diabetes, iPSC-derived pancreatic beta cells must not only produce insulin but also respond appropriately to changes in blood glucose levels. Ensuring that these cells function properly after transplantation is critical to the success of iPSC-based gene therapy.
Future Prospects And Conclusion
iPSC-based gene therapy holds great promise, with ongoing research aimed at overcoming current challenges and expanding therapeutic applications. Advances in CRISPR/Cas9 gene editing and tissue engineering are expected to further improve the precision and effectiveness of iPSC-based therapies. In addition, the development of 3D organoids derived from iPSCs offers new possibilities for modeling diseases and testing drugs in patient-specific settings.
As more clinical trials are initiated and safety issues are addressed, iPSC-based gene therapy is likely to play an increasingly important role in personalized medicine. Its potential in treating genetic diseases, cancer, and age-related diseases represents a major step forward in regenerative medicine, offering hope to millions of patients worldwide.
In summary, while challenges such as safety, immune rejection, and differentiation efficiency remain, the potential benefits of iPSC-based gene therapy are enormous. With continued innovation and clinical progress, iPSCs will revolutionize the field of gene therapy and provide new avenues for treating previously incurable diseases.
References
- Shinya Yamanaka, Induced pluripotent stem cells: past, present, and future. Cell Stem Cell 2012; 10: 678-84. (DOI: 10.1016/j.stem.2012.05.005)
- Jonas Cerneckis et al., Induced pluripotent stem cells (iPSCs): molecular mechanisms of induction and applications. Signal Transduction and Targeted Therapy 2024; 9: 112. (https://doi.org/10.1038/s41392-024-01809-0)
- R Grant Rowe and George Daley, Induced pluripotent stem cells in disease modelling and drug discovery. Nature Reviews Genetics 2019; 20: 377-88. (https://doi.org/10.1038/s41576-019-0100-z)
- Giulia Paolini Sguazzi et al., Induced pluripotent stem cells (iPSCs) and gene therapy: a new era for the treatment of neurological diseases. International Journal of Molecular Sciences 2021; 22: 13674. (DOI: 10.3390/ijms222413674)
- Sandra Petrus-Reurer et al., Immunological considerations and challenges for regenerative cellular therapies. Communications Biology 2021; 4: 798. (https://doi.org/10.1038/s42003-021-02237-4)
- Mourad Aboul-Soud et al., Induced pluripotent stem cells (iPSCs)-roles in regenerative therapies, disease modelling and drug screening. Cells 2021; 10: 2319. (DOI: 10.3390/cells10092319)
- Stefano Biressi et al., Stem cell therapy for muscular dystrophies. Journal of Clinical Investigation 2020; 130: 5652-64. (DOI: 10.1172/JCI142031)
- Selami Demirci et al., Gene therapy for sickle cell disease: an update. Cytotherapy 2018; 20: 899-910. (DOI: 10.1016/j.jcyt.2018.04.003)
- .Andrew Berical et al., A multimodal iPSC platform for cystic fibrosis drug testing. Nature Communications 2022; 13: 4270. (https://doi.org/10.1038/s41467-022-31854-8)
- Lubos Danisovic et al., Induced pluripotent stem cells for Duchenne Muscular Dystrophy modeling and therapy. Cells 2018; 7: 253. (DOI: 10.3390/cells7120253)
- Matin Chehelgerdi et al., Exploring the promising potential of induced pluripotent stem cells in cancer research and therapy. Molecular Cancer 2023; 22: 189. (DOI: 10.1186/s12943-023-01873-0)
- Robert Sterner and Rosalie Sterner, CAR-T cell therapy: current limitations and potential strategies. Blood Cancer Journal 2021; 11: 69. (DOI: 10.1038/s41408-021-00459-7)
- Muhammad Sadeqi Nezhad et al., Induced pluripotent stem cells (iPSCs) provide a potentially unlimited T cell source for CAR-T cell development and off-the-shelf products. Pharmaceutical Research 2021; 38: 931-45. (DOI: 10.1007/s11095-021-03067-z)
- Michael Xavier Doss and Agapios Sachinidis, Current challenges of iPSC-based disease modeling and therapeutic implications. Cells 2019; 8: 403. (DOI: 10.3390/cells8050403)
- Maria del Carmen Ortuno-Costela et al., The challenge of bringing iPSCs to the patient. International Journal of Molecular Sciences 2019; 20: 6305. (DOI: 10.3390/ijms20246305)
- David Paik et al., Patient and disease–specific induced pluripotent stem cells for discovery of personalized cardiovascular drugs and therapeutics. Pharmacological Reviews 2020; 72: 320-42. (DOI: 10.1124/pr.116.013003)
- Cristina Marisol Castillo Bautista and Jared Sterneckert, Progress and challenges in directing the differentiation of human iPSCs into spinal motor neurons. Frontiers in Cell and Developmental Biology 2022; 10: 1089970. (DOI: 10.3389/fcell.2022.1089970)
About The Author:
Jack (Jie) Huang, MD, Ph.D., is the chief scientist and founder/CEO at CSTEAM Biotechnology in Ohio, USA. He is also recognized as a medical science writer, an inventor, and a STEM educator. Huang completed his MD/Ph.D. at Shiga University of Medical Science (SUMS) in Japan in 2000. He then pursued postdoctoral training in immunology at the University of Tokyo, followed by a role as research assistant professor at Tohoku University. In the U.S., he served as a postdoctoral fellow at Oregon Health & Science University and as an oncologist and senior research scientist at Ohio State University Nationwide Children’s Hospital, focusing on oncology research until 2018, when he launched his company specializing in biological models and biochips. Additionally, Huang was vice president of the American Botanical Drug Association, director of the Stem Cell Engineering and Technology Research Center at the Industry Technology Research Institute of Chongqing University, and chief scientist of the International Institute of In Vitro Diagnostics at Chongqing Medical University. His research interests, which span genetic engineering biological models, gene-edited stem cells, immune cell drugs, and biochip technology, have led to numerous patents.