Cost-Effective Biomanufacturing Of Lentiviral Vectors
By Aziza P. Manceur, Martin Loignon, and Rénald Gilbert
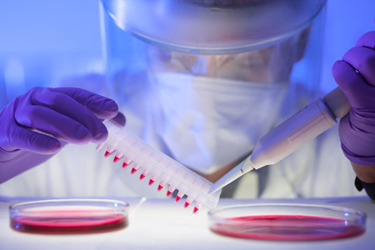
Lentiviral vectors (LVs) are proficient vectors for transferring genetic material in host cells for R&D and clinical applications, which include correction of immunodeficiencies and blood disorders and use in CAR T cell therapies. For the latter, LVs are used to engineer cancer patients’ own T cells to increase their capacity to detect and destroy cancer cells. Six FDA-approved products have reached the market in the past five years at prices ranging from $373,000 to $475,000 (Kymriah, Yescarta, Abecma, Breyanzi, Tecartus, and Carvykti). The significant cost of manufacturing LVs used to engineer patients’ T cells is part of an elaborate chain of supply that explains the high price; by improving the LV manufacturing processes, we can increase the cost-effectiveness of these therapies.
Here, we compare the fundamentals of current and upcoming LV production processes and highlight areas of improvement that would allow an increase in biomanufacturing cost efficiency. First, the current method of production using transfection could be revisited, as it seems to have reached its limit in terms of cost reduction, whereas new alternatives such as stable producer cell lines show promise to significantly lower operational expenses. Second, the cellular platform must be carefully selected, as it has a significant impact on the entire upstream process. For example, choosing adherent or suspension cells will have considerable consequences on the costs of scale up and manufacturing. Third, given LVs’ labile nature, an adapted perfusion process for production could offer an advantage over the more commonly used batch mode. During perfusion, fresh medium is continuously added to the culture, supplying nutrients and removing waste. This process supports higher cell viability and decreases the residence time of LVs in the vessel, thereby increasing the number of infectious particles produced.
These three strategies are summarized in Figure 1 and Table 1 and are described in more detail below.
Figure 1: Venn diagram of factors influencing the cost of LV biomanufacturing. Cost-effective productions will emerge from combinations and continuous improvement of platforms, production methods, and bioprocesses.
Table 1: Summary of production platforms and associated costs.
*A perfusion process applied to transfection was previously published.1
** Adherent packaging cells and adherent producer cell lines have also been published. Their advantages would be the same as suspension packaging and producer cell lines, except for the scalability limitation associated with adherent cells.
Production Method: Four-Plasmid Transfection Versus Stable Producer Cell Lines
Currently, LVs used in clinical settings are produced by transfecting cells with four plasmids. Three plasmids encode Gag/Pol, VSV-G, and Rev, genes that are essential for the structure and function of LVs. A fourth plasmid encoding the sequence of the gene of interest is added to the transfection mix. The main advantages of opting for transfection for LV manufacturing are rapidity and versatility. This process is appropriate when developing a portfolio that includes several target genes, or for optimizing gene sequences, as transfection offers the flexibility to quickly switch from one transgene to another. Nonetheless, transfection also comes with several constraints. The hefty production costs are driven by the price of transfection reagents and GMP-grade plasmids needed for producing clinical materials. The procurement of GMP-grade plasmids, high in demand, delays lead times by several months and has become a bottleneck of concern for biomanufacturing. Finally, with transfection being a stochastic event, the reproducibility from one production to another in terms of titers and LV quality is quite variable.
To address these issues, at the National Research Council Canada, we have generated a stable LV packaging cell line (PacLV-3D4) that can be used to produce LVs by transfecting one plasmid only (corresponding to the gene of interest) or to generate stable inducible producer cell lines. To engineer the LV packaging cell line, the LV accessory genes were stably integrated into the host genome under the control of a tight inducible system2. This inducible system is regulated by two molecular switches that directly and indirectly control the expression of LV cytotoxic elements upon the addition of two small molecules. Indeed, tight regulation is essential because the VSV-G protein and a protease coded by Gag/Pol are cytotoxic to mammalian host cells. The development of a packaging cell line has helped significantly reduce the cost since only one GMP-grade plasmid is needed instead of four for LV production.
Nevertheless, with packaging cell lines for lentiviral vectors, the gene of interest still has to be introduced by transfection. In order to forego completely the need for plasmids or transfection reagents, stable producer cell lines can be generated from packaging cells by stably transfecting the plasmid encoding the gene of interest. The main shortcoming of stable producer cell lines is the six-month period it takes to generate them. This necessitates the generation of a stable cell pool, followed by clonal selection and full characterization of individual clones that include stability, optimization of induction, titer measurement, and overall robustness. The generation of a GMP cell bank for the production of clinical materials requires an additional six months’ time.
In summary, the four-plasmid transfection is the most expensive system. Stable producer cell lines are the most cost-effective alternative since costly GMP-grade plasmids and transfection reagents are not required. However, if time is of the essence, packaging cell lines for lentiviral vectors offer the flexibility of the four-plasmid transfection at a lower cost since only one plasmid is transfected, with shorter timelines than the stable producer cell lines.
Cellular Platform: Suspension Versus Adherent Cells
Currently, LV manufacturing is frequently performed using adherent HEK293 cell lines grown in media with or without serum and transfected with four plasmids, even though this process is labor-intensive and poorly scalable. For clinical studies on small cohorts, this methodology to produce LVs in T-flasks, cell stacks, and other flatware may be satisfactory. For larger quantities, improvements in fixed-bed bioreactors have filled a gap in LV manufacturing using adherent cells, but scalability and costs are still limiting. As an example, based on our estimates, a 70 L production using a fixed-bed bioreactor is comparable to a 200 L production using suspension cells in terms of titer. The largest fixed-bed bioreactor growth area available is currently 500 m2 (fixed-bed volume of 60 to 70 L), limiting scale-up with adherent cells. With suspension cells, on the other hand, the process could be scaled up to the 2,000 L scale if needed. In addition, disposable bags for a 200 L production cost eight times less than the disposable unit required for a fixed-bed bioreactor. Further, issues related to cell distribution heterogeneity within fixed-bed bioreactors have been reported and bring additional challenges from a bioprocessing point of view.
Therefore, we would encourage clinicians and researchers to opt for a suspension system early in their development. At the NRC, we have developed a suspension HEK293 cell line (HEK293SF-3F6) that grows in serum-free conditions and can be used for producing LVs using four plasmids, but other HEK293-derived suspension cell lines are also available. In addition, we have generated an LV packaging cell line and stable producer cell lines that also grow in suspension in serum-free conditions.
Bioprocessing: Batch Versus Perfusion Mode
LVs produced in a bioreactor in batch mode are harvested at the very end of the process without replacing or adding fresh media. As an alternative, a fed-batch process has the potential to increase titers without the complexity of setting up a perfusion system. Similar to a batch process, the harvest is collected once at the end of the production period. However, the key is to find the right feed and regimen.3
A third option in terms of the upstream process is perfusion. During perfusion processes, fresh media are supplied continuously before and after production is initiated and LV-containing media are collected at defined intervals depending on the perfusion rate. Perfusion was developed in part to support cell viability during biologics production, which was found, in most cases, to also increase viral titers. This is due to the continuous supply of fresh nutrients and elimination of waste, limiting nutrient depletion and detoxifying the bioreactor environment. For labile LV particles, it also means that they are removed from a harmful environment, allowing better recovery of infectious particles.
In principle, a smaller footprint is needed since a smaller bioreactor can be used in comparison to batch modes. For instance, for a four-day process, four harvests can be collected in perfusion mode (assuming a perfusion rate of one vessel volume per day), whereas only one harvest is collected at the end of the production period in batch mode. Yet, the main drawback of working in perfusion is the higher level of complexity to set up and operate compared to batch mode, since multiple pumps, balances, and connectors are required, as well as a cell separation device and its controller. Other considerations include cold room space for the culture media and perfused harvest. However, benefits such as higher quality products and higher overall output outweigh the disadvantages. For instance, we found that the total cumulative titer was increased by tenfold in perfusion compared to a batch with a model LV expressing GFP in a 3 L bioreactor using our in-house producing cell lines.4
A key component in perfusion is to use a cell retention system that retains the cells in the bioreactor and lets the LVs pass into the harvest for collection. Systems that have been reported for such applications include the Viral Harvest Unit (Artemis Biosystems), the Cellicon Perfusion Solution (Millipore), the TFDF technology (Repligen), and the BioSep (Applikon, Getinge). Note that the BioSep is the only reusable system, while the other technologies are membrane-based and single-use.
Finally, downstream processing (DSP) is directly impacted by the mode of production. Typically, the harvest is clarified with filters to remove cells or cell debris, followed by a capture step by chromatography, concentration, and sterile filtration. Perfusion with membrane-based technologies results in less turbidity and lower cell by-products in the harvest than in batch mode. The clarification step should therefore be less complex, if needed at all, in perfusion mode. The main question, however, is whether each daily harvest should be treated as a different batch, or if all the harvests can be pooled together and processed as one batch in the next purification step, which is usually chromatography.
Another option would be to have a continuous capture step at the exit of the bioreactor, but this approach has not been successfully demonstrated yet for lentiviral vectors. Having rapid, affordable in-process analytical methods and tools will help in making these decisions quickly. The ability to measure viral titers during production is key to accelerating process development. A few methods, such as IEX-HPLC,5 the AlphaLISA, and flow virometry, provide viral titer estimates, but more rapid and precise analytical tools are still needed.
Conclusion
Given the cost and the limitations of using adherent cells, opting for a suspension cell line for LV manufacturing early in the development of a candidate will facilitate the scale-up and overall biomanufacturing process and reduce the cost, as shown in Table 1. The evaluation of candidates and lead optimization done at a small scale using transfection to generate LV candidates is fast and will indicate how efficacious the product is in a timely manner. As soon as the lead candidate is selected and Phase 1 clinical trials are initiated, a stable producer could be foreseen if a large enough number of patients are seeking treatment. Bridging studies between LV produced by transfection compared to LV produced with a stable producer can be performed before moving to Phase 2/3 studies. The availability of a generic LV packaging cell line provides a head start for developing producer cell lines and should supplant the four-plasmid transfection process as it has the potential to significantly reduce costs and timelines for LV manufacturing.
In terms of upstream bioprocessing, several options are now available to efficiently separate the cells from the LV product when working in perfusion mode. At the moment, CDMOs able to produce biosafety level 2 viral vectors in perfusion mode are scarce. While perfusion is a rather complex process, the increasing demand for LV and the potential of perfusion to increase recovery of infectious LVs, labile in nature, should drive its development and find more adopters.
CAR T cell therapies have shown great promise to treat aggressive and refractory cancers and, with their applications expanding, there is a growing need for high-quality LVs. CAR-Ts are highly personalized therapies developed through several manufacturing steps and complicated logistics, which explain the high cost. Reducing the cost of lentiviral vector biomanufacturing will enhance cost efficiency, leading to a decreased overall price of therapies and improved accessibility for more patients.
References
- Sven Ansorge, Stéphane Lanthier, Julia Transfiguracion, Yves Durocher, Olivier Henry, Amine Kamen, Development of a scalable process for high-yield lentiviral vector production by transient transfection of HEK293 suspension cultures, J Gene Med, 2009 Oct;11(10):868-76
- Broussau S, Jabbour N, Lachapelle G, Durocher Y, Tom R, Transfiguracion J, Gilbert R, Massie B. Inducible packaging cells for large-scale production of lentiviral vectors in serum-free suspension culture. Mol Ther. 2008 Mar;16(3):500-7.
- Chun Fang Shen,· Sonia Tremblay, Catherine Sabourin‑Poirier, Elodie Burney, Sophie Broussau, Aziza Manceur, Anja Rodenbrock, Robert Voyer, Martin Loignon, Sven Ansorge, Rénald Gilbert. Culture media selection and feeding strategy for high titer production of a lentiviral vector by stable producer clones cultivated at high cell density, Bioprocess and Biosystems Engineering, 2022 Aug;45(8):1267-1280
- Manceur AP, Kim H, Misic V, Andreev N, Dorion-Thibaudeau J, Lanthier S, Bernier A, Tremblay S, Gélinas AM, Broussau S, Gilbert R, Ansorge S. Scalable Lentiviral Vector Production Using Stable HEK293SF Producer Cell Lines. Hum Gene Ther Methods. 2017 Dec;28(6):330-339
- Julia Transfiguracion, Michelle Yen Tran, Stéphane Lanthier, Sonia Tremblay, Nathalie Coulombe, Mauro Acchione, Amine A Kamen. Rapid In-Process Monitoring of Lentiviral Vector Particles by High-Performance Liquid Chromatography. Mol Ther Methods Clin Dev. 2020 Aug 5;18:803-810.
About The Authors:
Aziza Manceur joined the National Research Council Canada as a research officer in 2013 and is part of the Cell Culture Scale Up team. Her current focus is on the optimization of biomanufacturing processes in order to improve the titer and the quality of lentiviral vectors. She obtained her Ph.D. in biomedical engineering from the University of Toronto and completed a postdoctoral fellowship at the University of Pennsylvania.
Martin Loignon joined the Human Health Therapeutics Research Centre of the National Research Council Canada in 2015 as the team lead of the Cell Engineering team. In this role, he has led projects related to the production and purification difficult-to-express recombinant proteins and contributed to the development of a CHO expression platform. Since 2020, he is leading the Cell Culture Scale-Up team, overseeing experts in bioprocess development and scale-up, and engineering viral-based and non-viral tools for gene editing. He holds a Ph.D. in molecular biology from Université de Montréal and a postdoctorate from McGill University. He has four years of experience at PerkinElmer-Biosignal in developing antibody- and cell-based assays for high throughput screening.
Rénald Gilbert is a senior researcher at the Human Health Therapeutics Research Centre of the National Research Council Canada (NRC). Throughout his career at the NRC, he has held leadership roles such as being the leader of the Vaccines & Immunotherapeutics program and the Biomanufacturing Research Initiative. Currently, he leads the Viral Vector Production team. He is also an adjunct professor in the Department of Bioengineering of McGill University. His research interests include the optimization of viral vectors for cell and gene therapy applications and the development of vaccines, as well as their methods of production. He completed his Ph.D. in biochemistry at McMaster University in 1994.