Challenges In Analytical Development And Testing For Gene Therapies
By Stephen Gacheru, Ph.D., Vice President, Analytical Development and Technical Operations at Center for Breakthrough Medicines
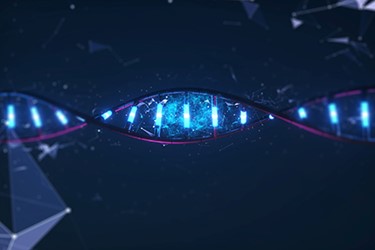
Given the rapid emergence of gene therapies based on recombinant adeno-associated virus (rAAV), there is a great need for appropriate analytical tools to support every lifecycle stage of these products.
Note that in this article “rAAV” refers to generic therapeutic constructs involving recombinant adeno-associated virus, regardless of serotype.
The FDA’s 2004 Process Analytic Technology (PAT) guidance outlined the agency’s vision for analytics as key indicators of product quality and safety. PAT emphasized the importance of in-process monitoring, thus exceeding the standard at the time of relying mostly on end-product testing. This practice, known as “testing-in” quality, is still widely used but considered outdated. Since the mid-2000s FDA and European regulators have instead promoted Quality by Design (QbD), an approach that assures quality at every stage of production while providing flexibility in addressing quality issues as they arise. For therapeutic proteins QbD still lags behind testing-in quality. This is partly due to the established nature of therapeutic protein manufacture, whose economics can tolerate occasional batch failures. The more complex nature of cell-based therapy manufacturing, the higher value involved, and the more dire consequences of batch failure suggests that QbD approaches will be considered more comprehensively and from the earliest development stages.
With gene therapies the viral vector is arguably the most critical component, and therefore the one whose manufacture is in the greatest need of in-process analysis. Lentivirus and AAV, currently the most used viral vectors, are extremely well characterized but their complexity and potential variability during production presents challenges for both end-product and in-process analytics.
Clearly, producing the safest, most consistent, and highest-quality gene therapy products demands development of robust, scalable, optimized production and purification strategies. Those goals are not achievable without similarly optimized analytics for monitoring key quality attributes (CQAs) related to the product’s physical integrity as well as its therapeutic objectives.
The Analytical Strategy
The wide range of analytical methods for assessing rAAV product quality reflects the complexity and diversity of cell therapy products. Developers must comply with current Good Manufacturing Practices, described in applicable sections of 21CFR211 and 21CFR610[1], for example, to ensure identity, dose, potency, purity, and safety. Specific (if somewhat dated) guidance for identifying, developing, and validating analytical methods to support cell and virus bank characterization, final-product release, and stability studies may be found at the Center for Biologics Evaluation and Research (CBER)[2], and in various ICH guidelines.
The specific strategy for both upstream and downstream testing involves first establishing In-process specifications, then testing for those characteristics to confirm that the manufacturing process is controlled, consistent, and aseptic.[3]
Optimizing upstream and downstream production for rAAV, while emphasizing quality, requires thorough characterization of critical quality attributes (CQAs). For rAAVs virus titer, capsid content, and capsid aggregation are the CQAs most likely to affect the therapeutic product’s potency, purity, and safety.[4]
Traditional analytical methods for quantifying these CQAs are time-consuming, low-throughput, and unsuited for the fast-paced process development. Generally, these analytical approaches, which were developed for R&D settings, must be re-evaluated and rigorously validated to support clinical trials. Moreover, assays suited to phase 1 trials may require modification as clinical development proceeds. This process is both iterative and continuous.
Additionally newer, more streamlined assays are emerging, but experience in both industrial and academic labs is limited. Ultimately, the goal is rapid, real-time at-line characterization to avoid the delays and potential errors involved in taking samples at the production site and analyzing them remotely.
Early Development
Drug development begins with analytical strategies applicable to control strategies across the product-process lifecycle. Experience with these methods gained during early stages -- “discovery” on the product side and “preclinical” for material requirements -- is applied to subsequent stops along the value chain, up to finalization of the manufacturing processes for drug substance and product.
During preclinical studies companies focus on safety-related analytics to support the therapy’s Investigational New Drug (IND) application. Tentative safety profiles are confirmed during first-in-human trials.
However, at this stage not much is known about process- and product-related impurities that may present safety issues. Thus, at this stage analytical tools should identify impurity levels that would trigger the need for in-process-control (IPC) tests. These in turn establish the molecule’s critical quality attributes (CQA) that eventually become the process control elements driving the drug substance’s final specifications. These analytical tests, as part of process controls, are used to set limits or alerts that are eventually adopted for large scale manufacturing.
As process development moves to larger scales to accommodate clinical programs the analytical tools, particularly those related to potency and impurity profiles, are continuously improved for greater robustness. The major concern here are side products of the manufacturing process, specifically unfilled or partly filled capsids.
Due to a lack of established reference standard materials (RSMs) against which to assess analytical tools, challenges to establishing an analytical strategy first emerge during process development.
Having appropriate rAAV RSMs in hand is critical for having points of reference for many CQAs, including for particle titer, vector genome titer, and infectious titer[5]. Along with RSMs, developers need extremely accurate titration methodology, once RSMs are in place, to provide consistency in aliquoting dosages. RSMs are currently available commercially for rAAV serotypes 2 and 8, which reflects usage of these serotypes in current clinical development. Most trials use rAAV2[6], but interest in other serotypes is growing. Investigators are interested in serotype 8 because its tropism, distinct from that of serotype 2, allows targeting different cells and tissues for transduction. Note that the World Health Organization offers a lentiviral RSM, which it offers to researchers for free.
The poor lab-to-lab reproducibility in application of existing RSMs has been a major obstacle to standardizing product activity and dosage potency, and to establishing uniformity standards for laboratory-specific standard materials and protocols.[7]
With RSMs in hand, researchers apply standard molecular analyses to characterize rAAV preparations, for example optical density, qPCR, or ELISA for virus titer, electron microscopy or analytical ultracentrifugation for content, and light scattering methods to quantify levels of aggregation.[8]
Potency Assays
Initial potency assays supporting efficacy studies typically rely on established drug interaction and binding studies. As process development advances, the need may arise to enhance potency to capture fully the drug’s potential in the intended disease. For cell and gene therapy products, these assays may require additional cell line development specific to each product and, sometimes, a comparison of assay performance in vivo and in vitro. Engineering cell lines for specific functional potency remains a challenge for gene therapy, as it requires knowledge of disease pathogenesis.
The FDA recommends that potency assays include in vitro and/or in vivo tests as well as full characterization of the biological processes and that this be done early in development to assure identity, quality, purity, potency, and stability. Developing in vitro potency assays for gene therapy products includes at least two steps: demonstrating the transfer genetic material and showing that the transferred genetic material has the desired effect -- typically production of a functional protein. Potency assays should be quantitative and expressed in units of activity calibrated against recognized standard or reference standard from a reliable source.
Several challenges exist in developing potency assays tests for cell and gene therapy products:
- inherent variability of starting materials
- limited lot size and materials for testing
- limited stability
- lack of appropriate reference standards
- interactions between multiple active ingredients (vectors containing multiple genes)
- complex modes of action involved in both the disease and treatment (e.g. infection, integration, and expression of transgene), and the in vivo fate of the product as it relates to migration from the administration site, cellular differentiation, viral infection, and transgene expression
Gene therapy products using AAV vector-based delivery currently undergo in vitro and in vivo potency assays, for the specific mechanisms of action under investigation, at the DNA, RNA and protein levels. These are mostly standard assays based on ddPCR, qPCR, western blots, ELISA, immunohistochemistry, etc.
The functional potency assay matrix includes cell line and animal model selection and/or development specific to the particular gene therapy drug and its promoter, viral transducibility and infectivity, tissue selectivity of AAV serotype(s), dose-ranging studies, activity assays (e.g. enzymatic activity), the emergence of common impurities during sample processing, and assaying for relative potency against a reference material.
The objective is confidence in our ability to develop and optimize assays specific to a specific investigational drug, used for a specific disease and delivered in a specific way.
The Center for Breakthrough Medicines approaches potency assays for gene therapies through a "matrix" approach that involves capturing two or more mechanisms of action to compile data with the goal of reaching a collective conclusion regarding final product potency. Our strategy involves the following assays:
- Virus product strength by ddPCR and infectivity through ddPCR. We have developed platform assays for these two techniques which we are now preparing for validation.
- Translation Potency via ddPCR. By following expression of the gene of interest to the mRNA stage, we can detect mRNA as part of early development potency assays.
- Expression of polypeptide chains via ELISA, using a representative green fluorescent protein sample analysis to set up a template assay design.
- Functional protein potency assay through ELISA, based on disease mechanism of action (MOA).
Product Characterization Studies
Characterization studies for Cell and Gene Therapy products are more challenging than what one would expect for a conventional protein biologic.
The main issue is the inclusion of product-related impurities arising from the non-uniform DNA incorporation into the viral capsid. Additionally, variously partially full as well as empty capsids are generated during manufacturing. It remains unclear whether these product-related impurities are immunogenic or present any risks to patients. However, prudence dictates that these impurities be reduced as much as possible or tested for possible immunogenicity or other toxicities.
Moreover deamidation[9], oxidation[10], and glycosylation[11] influence the potency and activity of these side-products. These modifications could lead to aggregation of the viral particles, another potential source of immunogenicity. The extent this occurs is unclear, but full characterization of these proteins should lead to better understanding of how to improve the safety and efficacy of these products.
The analytical tools employed for characterizing capsid proteins form the basis of comparability studies to support scaleup and process improvements. They can also provide information towards understanding the stability-indicating capability of these analytical tools and thus lead to improved formulations of rAAV-based gene therapy products.
Impurities and Degradation
Since rAAV therapies are so novel, investigators must investigate every avenue for potential quality issues related to product instability. For example, peptide oxidation is a major cause of chemical and physical instability in protein therapeutics, so it comes as no surprise that oxidation is implicated in rAAV product safety and quality as well. Capsid proteins are known to oxidize on exposure to light and through the interaction between product and metal ion impurities in raw materials and excipients.[12] Minimizing these effects involves a thorough characterization of metal impurities in those ingredients, as well as deploying free amino acid scavengers such as methionine or histidine, plus well-characterized metal ion scavengers such as ethanol, EDTA and DTPA.[13]
Achieving this objective, especially during later (downstream) production steps, is complicated by the presence of byproducts, host cell proteins, and DNA, whose concentrations may exceed that of the rAAV product by a factor of 100.[14] In a recent review of new and emerging methods for downstream rAAV process analytics, the authors compare established and newer methods based on turnaround time, preparation, required volumes, repeatability, etc.[15]
A further issue related to analytical development arises from the need to scale rAAV-related products to meet global demand and the subsequent storage and transportation of these therapies. Reduced product quality over time, resulting in lower yield and purity and shorter shelf-life compared to recombinant protein therapeutics, underscores the need to understand how to preserve CQAs throughout manufacturing, storage, and shipment through rigorous characterization of degradation mechanisms and the development of formulation strategies to combat those processes.[16]
The degradation mechanisms involved are already familiar: stresses resulting from temperature excursions, shear, freezing-thawing, and light exposure. Mitigating these effects through optimization of buffers, pH, excipients, and storage protocols has been the focus of recent development efforts. We expect that a deeper, more comprehensive understanding of the mechanisms through which these factors operate, plus knowledge of how impurities affect product quality, will go a long way towards achieving the efficiencies, safety, and confidence stakeholders currently experience and expect from legacy biologic therapies.
- https://www.accessdata.fda.gov/scripts/cdrh/cfdocs/cfcfr/CFRSearch.cfm?CFRPart=211
- https://www.fda.gov/regulatory-information/search-fda-guidance-documents/guidance-human-somatic-cell-therapy-and-gene-therapy
- https://link.springer.com/protocol/10.1007%2F978-1-61779-370-7_17#citeas
- https://www.sciencedirect.com/science/article/pii/S2329050121000255
- https://pubmed.ncbi.nlm.nih.gov/25275822/
- https://www.cell.com/molecular-therapy-family/molecular-therapy/fulltext/S1525-0016(16)32457-1
- https://www.ncbi.nlm.nih.gov/pmc/articles/PMC2957240/pdf/hum.2009.223.pdf
- https://www.sciencedirect.com/science/article/pii/S2329050121000255
- https://www.sciencedirect.com/science/article/pii/S1525001618304544
- https://bmcbioinformatics.biomedcentral.com/articles/10.1186/s12859-017-1848-9
- https://www.ncbi.nlm.nih.gov/pmc/articles/PMC7496479/
- https://www.sciencedirect.com/science/article/pii/S002235491930735X
- https://www.sciencedirect.com/science/article/abs/pii/S0022354915504176
- https://www.ncbi.nlm.nih.gov/pmc/articles/PMC5423478/
- https://www.cell.com/action/showFullTableHTML?isHtml=true&tableId=tbl4&pii=S2329-0501%2821%2900025-5
- https://link.springer.com/protocol/10.1007%2F978-1-61779-370-7_17#citeas