Beyond CRISPR: Choosing The Right Gene Modification Technology For Mouse Model Generation
By Kenneth Albrecht, Ph.D.
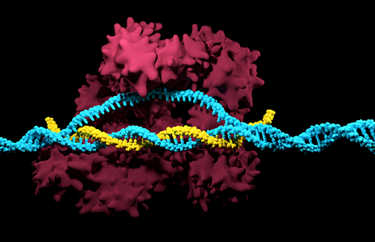
It’s difficult to discuss the genetic modification of animal models without CRISPR entering the conversation. While technologies like CRISPR/Cas9 have extended the boundaries of what is feasible for model development — increasing the speed and efficiency with which genetic modification can be accomplished, while reducing costs — the excitement and promise associated with CRISPR can create the misperception that it is always the best tool in the toolbox. This misperception can cause confusion, concern, or frustration for researchers who need a genetic modification for which CRISPR may not be the best approach.
The fact is, there are a wide range of genetic modification technologies and approaches, each with its advantages, drawbacks, and appropriate applications. A review of these options can help bring clarity and provide guidance on which approach may be best suited to a particular model generation project, ensuring that the selected approach is not only technically feasible but capable of being done efficiently and practically in a production environment.
Gene Editing Via CRISPR
Starting with the technology that garners the most attention today, the CRISPR/Cas gene editing system is used to induce precise genetic alterations using a relatively simple protocol. The most significant advantage of gene editing via CRISPR is the relatively fast timeline to develop a new rodent model, a benefit that understandably drives many investigators to consider CRISPR when a genetically modified model is required.
The accelerated timeline is mostly due to the fact that CRISPR does not require the time-intensive effort of designing a gene targeting vector or manipulating embryonic stem (ES) cells. Instead, the mouse genome is accessed at the zygote stage of embryonic development by microinjection, making it possible to generate founder animals with the desired mutation quickly — averaging only 24 weeks to arrive at a small group of heterozygote mice. This timeline is significantly faster than approaches using gene targeting in ES cells, which in turn reduces the overall cost of the project and enables a study to commence sooner.
Another advantage of CRISPR is the flexibility to use it for genetic modification of rodents from a wide range of genetic backgrounds, because it is not dependent on the availability of ES cells. In theory it can be used with virtually any mouse line or strain — the caveat being that some lines (strains) are not good reproducers and therefore not likely candidates for model generation or breeding.
Though versatile and relatively fast, CRISPR is not ideally suited to all genetic modification projects, primarily due to limitations in the size and complexity of the genetic modifications feasible. Currently, the applications for which CRISPR is most efficient include point mutations, constitutive gene knockouts, and small genomic insertions. However, when it comes to the size of the genomic insertion, the upper limit for CRISPR is expanding rapidly. It is now also feasible to use this evolving technology for performing targeted transgenesis (e.g., inserting a murine or human cDNA of interest without interfering with other gene expression) and for generating knock-in models that express site-specific recombinases or reporters. However, more complex alleles (including large genomic replacements and conditional knock-ins) are likely better handled by an approach other than CRISPR, at least as of this writing.
An additional drawback of CRISPR (or any other nuclease-based gene editing technology) is the potential for off-target cleavage of the DNA. However, various analyses of mice generated using CRISPR/Cas suggest a lower incidence of such cleavage in vivo.
Gene Targeting Via Homologous Recombination
Perhaps the most proven genetic modification approach is gene targeting using homologous recombination in ES cells. In use for several decades, homologous recombination is well-suited to many applications, and the associated risks are well-known, well-documented, and fairly limited.
Due to its versatility and targeted nature, homologous recombination-mediated gene targeting remains the method of choice for complex genetic modifications. It is conducive to larger genetic insertions and more complex genetic modifications than CRISPR — for example, humanizing a mouse target gene by replacing it with its human counterpart or inserting a large genomic region. Homologous recombination via ES cells is also the method most often used for performing targeted transgenesis to develop a transgenic mouse, particularly when the transgene is large or relatively complex.
Though proven and versatile, homologous recombination does have drawbacks, chief of which is the associated timeline. On average, it takes 42 weeks for vector construction, ES cell targeting, chimeric mice production, and finally production of a small group of heterozygotes — a measurably longer timeline than gene editing. This typically correlates with a higher cost, as well as delays in moving a study forward.
Additionally, because homologous recombination requires the use of mouse ES cells, its application is limited to the availability of cell lines for the desired genetic background, which in turn is limited to the cell lines a given model generation company has access to and employs.
Random Integration Transgenesis
Random integration transgenesis, often performed using pronuclear injection (PNI), follows a process and timeline similar to CRISPR, but the genome modification event is the random insertion of an exogenous DNA segment.
Random integration transgenesis can be a useful approach when gene overexpression is desired — for example, to model DNA copy number amplifications associated with cancer — or simply to study a gene’s expression pattern by tagging it with a reporter.
The flexibility to perform random integration transgenesis on rodents of various genetic backgrounds, coupled with its relatively quick timeline, are key advantages. On the other hand, there is the potential for introduction of the unwanted mutation due to random transgene insertion, or for unintended epigenetic silencing to occur in successive generations.
Gene Modulation Via Short Hairpin RNA Interference
For studies in which it is desirable to analyze the effect of downregulating a target gene without deleting it from the genome, the use of gene modulation employing short hairpin RNA interference (shRNAi) is a good option. Though currently underutilized, this genetic modification technique appears to be coming back into favor, perhaps due to its flexibility.
shRNAi allows a targeted gene’s expression to be silenced in a specific and long-lasting manner; at the same time, the technique is inducible and reversible. These features make it possible to use a model generated using shRNAi to mimic the effects of an inhibitory drug and elucidate the drug candidate’s therapeutic profile more effectively than other genetic modification approaches may allow. For instance, a model in which the target gene is temporarily modulated may be conducive to studies of a drug’s dosage regimen.
One of the key drawbacks of shRNAi-based gene expression modulations is the potential for off-target effects. Thus, a well-designed targeting vector is important. Good design of shRNAs is key and testing of shRNAi target and potential off-target genes in vitro (in targeted ES cells) helps mitigate risk. A poorly designed vector can lead to misleading data, with the potential to sidetrack downstream efficacy studies.
Conclusion
Selection of the right genetic modification technique is an important consideration in generating a new animal model, and one that should be made based on full knowledge of the pros and cons of each available option. Careful consideration of these genome modification tools can ensure that the optimum approach is employed, thus putting you on the right track for successfully reaching the goals and objectives of the study.
About The Author:
Kenneth Albrecht is associate director of scientific program management at Taconic Biosciences and an adjunct assistant professor at Boston University School of Medicine. He has authored or co-authored over 25 scientific publications and has been engaged in mouse model generation and characterization for over 25 years.