Assessing Quality Of Viral Vectored Vaccines
By Diane McCarthy, USP
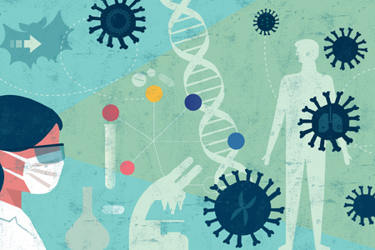
The COVID-19 pandemic has spurred rapid development and authorization of several new vaccine platforms, including mRNA and viral vectored vaccines.1 In October, I wrote about critical quality attributes and analytical procedures of mRNA vaccines. In this article, I focus on viral vectored vaccines.
While viral vectored vaccines have been previously developed against Ebola virus, the COVID-19 vaccines were the first widespread global application of this vaccine platform. As of September 2022, there are four COVID viral vectored vaccines that have received regulatory authorization in one or more countries and more than two dozen additional viral vectored COVID vaccines in clinical trials.2 The urgency to respond to the COVID-19 pandemic required rapid scale-up of manufacturing and development and implementation of quality control tests to ensure safe and effective vaccines. With multiple manufacturers around the world manufacturing viral vectored vaccines and with contract manufacturers playing a key role in expanding production capabilities, a wide variety of analytical tests have been established to assess vaccine quality. Many countries receiving vaccines approved in other countries bypassed in-country acceptance testing in favor of accepting testing results from the country of origin.
However, as the world moves to a more normal pace of vaccine development and distribution, it is important for global stakeholders to align on quality for viral vectored vaccines. Since the successful widespread application of this technology is relatively new, there are limited regulatory guidelines and industry standards to guide quality during development and manufacturing. To address the need, the United States Pharmacopeia (USP) has convened vaccine experts to identify important quality attributes for viral vectored vaccines and to propose test methods that can be used to assess the quality of these vaccine products. The resulting draft guidelines, Analytical Procedures for Viral Vectored Vaccine Quality, have been published for public comment and feedback. Below, I summarize the product quality attributes and proposed methods that can be used to ensure vaccine quality.
Quality Attributes Of Viral Vectored Vaccines
Viral vectored vaccines utilize a live virus that is engineered to produce a specific antigen when introduced into the patient. Adenovirus is the most commonly used virus, but modified vaccinia, measles, influenza, and vesicular stomatitis viruses have also been used for vaccines. There are at least 88 human adenovirus (Ad) types, with many capable of infecting a variety of dividing and non-dividing cells.4 The Ad genome is well characterized and can be engineered to render it replication-incompetent while introducing transgenes encoding the desired antigen that can only be expressed in appropriate cell types.5 Also, Ad vectors have been extensively tested as vaccine delivery systems in preclinical studies and clinical trials.6 There are currently six Ad vector-based vaccines approved or authorized for emergency use by global regulatory agencies, including two Ebola vaccines developed prior to the pandemic and four COVID-19 vaccines.
While some quality tests are similar to those for other vaccines (such as safety tests), adenovirus vectored vaccines have unique critical quality attributes (CQAs) that must be tested to ensure quality and safety of the vaccine (Table 1 below). Viral vectored vaccines have two major components: the DNA sequence encoding the antigen and the viral vector used as a delivery device (which includes the capsid as well as the genomic DNA to support infectivity), so testing of both components is necessary to confirm the identity of the vaccine. The sequence of the cargo DNA can be assessed using common nucleic acid techniques like quantitative polymerase chain reaction (qPCR) or next-generation sequencing.
Table 1. CQAs and Analytical Procedures for Viral Vectored Vaccine Quality.
aOnly tested in drug product
The identity of the adenovirus vector is determined by analyzing the structural components of the viral capsids, which are composed of three different viral proteins (VP1, VP2, and VP3) in varying stoichiometries.7 The ratio of these proteins is specific to different adenovirus subtypes, and measuring these ratios provides a way to ensure the identity of the viral capsid. Common methods for protein analysis, including reverse-phase ultra-performance liquid chromatography (RP-UPLC), liquid chromatography coupled to mass spectrometry (LC-MS), and immunoassay methods like Western blot can be used to establish the identity of the viral capsid.
Since viral vectored vaccines are produced in cell lines, process-related impurities can include residual host cell proteins (HCP) and host cell DNA (HCD) that have not been purified away during downstream unit operations. These protein and DNA residuals are typically monitored with quantitative ELISA and qPCR, respectively. Product-related impurities can also include the presence of empty capsids. These empty capsids lack the DNA encoding for the antigen and therefore should be minimized and excluded from the dose determination. Viral vectors are also prone to aggregation, which can reduce vaccine efficacy and increase safety risk. Aggregates can be monitored using various light scattering techniques such as dynamic light scattering (DLS).
Potency of viral vectored vaccines can be measured at several different levels, and typically multiple orthogonal methods are used to establish potency. Potency includes multiple steps such as infection, transcription, translation/protein expression, and immunogenicity. The first level of potency assessment is to establish that the viral vector has the capability to infect the cells in order to deliver the DNA cargo. Flow cytometry-based infectivity assays or TCID50 assays are typically used for this purpose. The level of transgene expression can also be used to evaluate potency. This is accomplished by means of a cell-based transgene-ELISA using a cell line that does not support vector replication.8 Dose, or infectious units, is measured using a quantitative PCR based potency assay performed in HEK293 cells. The mass measured by qPCR is assigned a level of activity by using a standard curve of replicated adenovirus genomes that has been correlated with infectious potency using a traditional end-point dilution assay.9 Finally, the number of physical virus particles is measured by qPCR, and the ratio of particles to infectious units is used to assess viral infectivity.
Building Consensus
Public standards serve as the foundation for a robust global quality environment that assists manufacturers by increasing predictability and reliability of their production processes. However, new vaccine platforms are burdened with a lack of consensus on CQAs, limited publicly available information on testing strategies, and a lack of standardized methods. This problem is compounded by the fact that vaccines are a broad and diverse group of technologies that require specific expertise for a given manufacturing process.
USP has worked to address these challenges in a stepwise manner, first developing the COVID-19 Vaccine Quality Assessment Toolkits to provide general information on analytical tests that are relevant for vaccines. The resource currently includes a toolkit that summarizes tests that are common across all vaccines as well as platform-specific toolkits for viral vectored, mRNA, inactivated, and protein subunit vaccines. Additional toolkits for DNA, VLP, and attenuated virus vaccines are currently under development.
As a second step, and in response to stakeholder feedback that emphasized a need for detailed methods, USP worked with vaccine experts to develop the draft guidelines Analytical Procedures for Viral Vectored Vaccine Quality. This resource describes CQAs that are relevant for viral vectored vaccines, and provides detailed test methods. USP is seeking feedback from stakeholders on the proposed methods and encourages submission of alternative methods and validation packages. Since the launch of the viral vectored vaccine draft guidelines in April 2022, USP has already received comments and methods from manufacturers, regulators, vendors, testing labs, and academic researchers worldwide, which will be evaluated for inclusion in a future version of the guidelines.
References
- Verdecia, M. et al. COVID-19 vaccine platforms: Delivering on a promise? Human Vaccines & Immunotherapeutics, 1-21, doi:10.1080/21645515.2021.1911204 (2021).
- COVID-19 - Landscape of novel coronavirus candidate vaccine development worldwide; https://www.who.int/publications/m/item/draft-landscape-of-covid-19-candidate-vaccines
- Azarpanah, H., Farhadloo, M., Vahidov, R. & Pilote, L. Vaccine hesitancy: evidence from an adverse events following immunization database, and the role of cognitive biases. BMC Public Health 21, 1686, doi:10.1186/s12889-021-11745-1 (2021).
- Dhingra, A. et al. Molecular Evolution of Human Adenovirus (HAdV) Species C. Sci Rep 9, 1039, doi:10.1038/s41598-018-37249-4 (2019).
- Zhang, W., Fu, J. & Ehrhardt, A. Novel Vector Construction Based on Alternative Adenovirus Types via Homologous Recombination. Hum Gene Ther Methods 29, 124-134, doi:10.1089/hgtb.2018.044 (2018).
- Kochhar, S. et al. Defining the interval for monitoring potential adverse events following immunization (AEFIs) after receipt of live viral vectored vaccines. Vaccine 37, 5796-5802, doi:10.1016/j.vaccine.2018.08.085 (2019).
- Condezo, G. N. & San Martin, C. Localization of adenovirus morphogenesis players, together with visualization of assembly intermediates and failed products, favor a model where assembly and packaging occur concurrently at the periphery of the replication center. PLoS Pathog 13, e1006320, doi:10.1371/journal.ppat.1006320 (2017).
- Jogler, C. et al. Replication properties of human adenovirus in vivo and in cultures of primary cells from different animal species. J Virol 80, 3549-3558, doi:10.1128/jvi.80.7.3549-3558.2006 (2006).
- Wang, F. et al. Using QPCR to assign infectious potencies to adenovirus based vaccines and vectors for gene therapy: toward a universal method for the facile quantitation of virus and vector potency. Vaccine 23, 4500-4508, doi:10.1016/j.vaccine.2005.04.023 (2005).
About The Author:
Diane McCarthy, Ph.D., is senior director, science and standards in USP’s Global Biologics Department. Over the past few years, Diane has led a team that focuses on development of new standards that can help address common bottlenecks in the biotechnology industry across diverse areas, including vaccines, monoclonal antibodies, and cell and gene therapy. She also oversees development and maintenance of documentary and physical reference standards for biologics. Prior to joining USP, Diane worked for several small CROs that focused on the use of mass spectrometry for characterization of biologics, host cell proteins, and biomarkers.