Advancing PSC-Based Therapies: The Application Of Non-Integrating Engineering Strategies To Improve Directed Differentiation
By Dr. Erin Kimbrel, Ph.D., President Research, Astellas Institute for Regenerative Medicine, Astellas Pharma Inc.
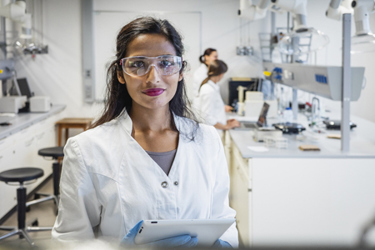
Allogeneic cell therapy products hold enormous potential to revolutionize disease treatment by facilitating the repair, replacement or rejuvenation of damaged cells and tissues. This innovative approach shows promise for patients with diseases for which there are few treatments or no cures. Pluripotent stem cells (PSCs) are at the forefront of these therapeutic strategies. PSCs serve as a replenishable starting material for the differentiation into a wide variety of therapeutically useful cell types, yet the inherent challenges in their directed differentiation present various hurdles that must be overcome for successful translation into clinically viable cell therapies.
Directed differentiation of PSCs involves mimicking developmental signaling cues ex vivo to guide them toward a specific cell type or tissue of interest. Most often, this involves a cocktail of cytokines, growth factors, and small molecules to stimulate or inhibit signaling pathways to specifically push cell fate toward a desired cell lineage and eventually to a mature cell phenotype. Despite the promising potential, challenges may arise within directed differentiation protocols. One challenge is the potential for cell differentiation to stall at a fetal-like stage, impeding the smooth transition to adult-stage cells. Another challenge is the lack of scalability during the differentiation process. A third challenge involves inefficiency of the process, where only a subset of cells faithfully transitions into the desired cell type, yielding a heterogeneous cell population which may dilute therapeutic potency or pose a safety risk.
To overcome these challenges, researchers are exploring a variety of techniques. Gene-editing technologies, such as CRISPR, offer a means to delineate cell fate through the introduction of specific and permanent modifications to cellular DNA. While gene-editing methods have been described in depth elsewhere, this article touches upon the prospective application of non-integrating strategies such as manipulating epigenetic signatures and utilizing RNA-based synthetic circuits to optimize the directed differentiation of PSCs. These non-integrating approaches, which avoid permanent genome modifications, significantly broaden the toolkit for developing cell therapies.
Mastering Cell Fate by Modifying the Epigenetic Landscape
In the intricate journey of cell differentiation, epigenetic mechanisms play a pivotal role in guiding stem cells from their embryonic origin to a matured cell type. These mechanisms involve heritable alterations that are unrelated to changes in the DNA sequence. Chemical changes to DNA, such as methylation, lead to gene silencing, while histone modifications such as acetylation, methylation and ubiquitination, influence chromatin structure. This dynamic interplay between epigenetic modifications and chromatin structural changes allows cells to maintain specific gene expression profiles and adapt to environmental cues or developmental signals.
Researchers can gain valuable insights by analyzing global chromatin accessibility, promoter occupancy, and methylation patterns across various gestational and postnatal stages. These insights can guide the development of methods to improve the maturation of PSC derivatives from fetal to adult stages and overcome obstacles in cell culture maturation. Advanced sequencing techniques like assay for transposase accessible chromatin (ATAC), in addition to established epigenomic techniques such as chromatin immunoprecipitation (ChIP) and bisulfite sequencing, enable mapping of the epigenetic landscape of cells in culture. This knowledge can be applied to stimulate specific epigenetic changes to induce gene expression alterations for improved cellular differentiation and maturation. For instance, the histone methyltransferase EZH1 serves as a negative regulator of lymphoid lineage maturation.1 Repressing its activity enables the maturation of αβ T cells, which may greatly facilitate the generation of mature T cells from PSCs in vitro. This could lead to the development of off-the-shelf allogeneic PSC-derived CAR T cell cancer therapies, thereby improving access to promising treatments that are currently only available as patient-specific autologous therapies. Another epigenetic regulator at the fetal-to-adult transition is the histone H2B ubiquitin ligase RNF20. This regulator plays a vital role in shaping the chromatin accessibility of key genes, influencing the postnatal maturation process of cardiomyocytes,2 which could help facilitate cell replacement strategies for ischemic heart damage following a myocardial infarction.
In addition to promoting cell maturation, epigenetic regulators can be used for the opposite purpose – to sustain cells in a proliferative progenitor state for a longer period, a feature that may be invaluable for scalability and/or in vivo engraftability of the cell product. For example, inhibiting the methyltransferase SetD7 retains the progenitor properties of muscle stem cells and improves the engraftment and repopulation ability of transplanted PSC-derived muscle cells. This is an important consideration for the development of cell-based treatments for congenital muscle diseases or following severe injury when substantial skeletal muscle reconstruction may be needed. Similarly, the human hematopoietic stem cell self-renewal agonist UM171 maintains expression of key self-renewal genes in hematopoietic progenitors, thereby substantially expanding the number of cells in culture prior to further differentiation into specific blood cell types, a feature that becomes important during large scale manufacturing of immune cell therapies.3
Overcoming Heterogeneity: PSC Purification Through RNA Switches and Sensors
Despite meticulous efforts to guide PSCs toward a desired mature state, the complexity of cellular responses often leads to a mix of cell types within the culture. This heterogeneity poses a significant obstacle, for example, when precise cell identity is crucial for potency, or the risk of unknown cell types poses a potential safety risk. Ensuring a consistent and known cell composition remains paramount in the development of cellular therapies for clinical use.
Innovative technologies such as RNA switches enable researchers to selectively control the fate of individual cells within the overall population and thus, can be used to address the challenge of heterogeneity. For microRNA (miRNA)-controlled switch technology, cell type-specific miRNAs serve as a sensor which can specifically bind to complementary sequences within a synthetic switch construct that also contains a transgene of interest. Depending on how the switch construct is set up, this binding can turn the encoded transgene 'on' or 'off'.4 For example, an ‘on’ switch encoding an apoptotic gene can induce programmed cell death in the subset of cells that express a particular miRNA sensor, promoting the elimination of that specific unwanted cell type.
Another RNA-based tool for improving PSC differentiation involves the exploitation of adenine deaminases acting on RNA (ADAR) enzymes, which can detect and repair mismatches in double-stranded RNA. In one version of this tool, termed “RADAR” for reprogrammable ADARs, a cell-state-specific RNA specifically binds to complementary guide RNA (gRNA) that also contains an in-frame “STOP” codon followed by a downstream transgene in a single synthetic construct. Double stranded RNA, formed through the binding of the gRNA and the cell-state specific RNA, triggers ADAR-mediated mismatch repair and removal of the in frame “STOP” codon to induce translation of the downstream transgene in a cell-state specific manner.5 In another version of this technology (the wonderfully named “DART VADAR” for Detection and Amplification of RNA Triggers Via ADAR), the downstream transgene encodes more ADAR machinery, effectively amplifying the RNA editing potential and allowing more dynamic control over transgene expression levels.6 RADAR and DART VADAR technology may both be useful tools to steer differentiation protocols more precisely, potentially through controlled expression of lineage-specific transcription factors or other factors mediating cell fate decisions, offering numerous possibilities to enhance the efficiency, purity, and/or maturity of PSC differentiation.
Streamlining Differentiation Protocols with Ribozyme-Controlled Transgene Circuits
Akin to ADAR-controlled RNA transgene expression systems, ribozyme-controlled synthetic circuits are being developed alongside Cas9/gRNAs to enable the spatiotemporal control of native gene expression but doing so without altering the genomic DNA. This innovative approach holds the potential to significantly reduce the duration of differentiation protocols by precisely timing the expression of key differentiation-inducing genes. Such a streamlined approach not only simplifies but also enhances the manufacturing process of cellular products.
In this method, ribozyme sequences are embedded within a gRNA sequence of a transiently transfected construct, preventing stable expression of the gRNA until a unique activating Cas9/ gRNA complex removes it. Once the ribozyme is removed, the active gRNA becomes stable and is able to pair with its complementary genomic DNA sequence. The gRNA also recruits a non-cutting Cas9-based transcriptional activator complex to the specific genomic DNA site which induces the upregulation of gene transcription. Sequential series of plasmids can be designed to activate in a specific order based on the target of each active gRNA. This enables the synthetic circuit to control the order and kinetics of expression for multiple genes of interest. Proof of platform experiments have shown that sequential daisy-chain circuits can complete their circuited expression patterns over the course of several days.7 While optimization is still necessary, synthetic gene expression circuits are an emerging tool that may add some level of sophistication for controlling multiplexed gene expression systems and accelerate differentiation without permanently modifying the genome.
Conclusion
The incorporation of non-integrating, traceless engineering tools in PSC directed differentiation approaches could significantly help streamline the development of allogeneic cell therapies, propelling regenerative medicine into a new era of possibilities. Astellas employs lineage-specific epigenetic modifications and innovative technologies to regulate cell fates, improve maturation, enhance purity, and streamline manufacturing. Crucially, these advancements can be achieved without introducing permanent changes to the cell's genome. This effort is part of a broader initiative8 focused on developing tools to enhance and expedite the creation of PSC-based cell therapies. Such advancements are pivotal in delivering effective cell therapies and creating scalable solutions that can successfully treat broader patient populations.
About the Author
Dr. Erin Kimbrel, Ph.D., is President of the Astellas Institute for Regenerative Medicine (AIRM), with over 25 years of extensive experience as a researcher and leader across diverse fields, spanning from molecular cancer biology to regenerative medicine. As the Head of AIRM, she offers strategic leadership and oversight for the development of cell therapy products across various therapeutic areas, including ophthalmology, immune-related disorders, vascular conditions, and liver disease.
Before assuming her role at AIRM, Erin held significant positions in the biotechnology sector. Notably, she served as the Senior Director of Development at Ocata Therapeutics and as Director of Research at Advanced Cell Technology. She is a co-author of over 25 publications and an inventor on numerous patents. For inquiries or collaborations, reach out to her at erin.kimbrel@astellas.com.
References
- Jing R, Scarfo I, Najia MA, Lummertz da Rocha E, Han A, Sanborn M, et al. EZH1 repression generates mature iPSC-derived CAR T cells with enhanced antitumor activity. Cell Stem Cell. (2022) 29:1181–96
- Lin CY, Chang YM, Tseng HY, Shih YL, Yeh HH, Liao YR, et al. Epigenetic regulator RNF20 underlies temporal hierarchy of gene expression to regulate postnatal cardiomyocyte polarization. Cell Rep. (2023) 42:113416.
- Chagraoui J, Girard S, Spinella JF, Simon L, Bonneil E, Mayotte N, et al. UM171 preserves epigenetic marks that are reduced in ex vivo culture of human HSCs via potentiation of the CLR3-KBTBD4 complex. Cell Stem Cell. (2021) 28:48–62.e6
- Fujita Y, Hirosawa M, Hayashi K, Hatani T, Yoshida Y, Yamamoto T, Saito H. A versatile and robust cell purification system with an RNA-only circuit composed of microRNA-responsive ON and OFF switches. Sci. Adv. (2022) 8:eabj1793
- Jiang K, Koob J, Chen XD, Krajeski RN, Zhang Y, Volf V, et al. Programmable eukaryotic protein synthesis with RNA sensors by harnessing ADAR. Nat Biotechnol. (2023) 41:698–707
- Gayet RV, Ilia K, Razavi S, Tippens ND, Lalwani MA, Zhang K, et al. Autocatalytic base editing for RNA-responsive translational control. Nat Commun. (2023) 14:1339
- Clark R, Terry AR, Pennington H, Hasty C, MacDougall, MS, Regan M, Merrill, BJ. Sequential Activation of Guide RNAs to Enable Successive CRISPR-Cas9 Activities. Mol. Cell (2021) 81: 226-238.
- Astellas DX Strategy Series Vol. 2: Drug Discovery Platform Integrating Humans, AI and Robots https://www.astellas.com/en/stories/dx_strategy_series_vol.2#:~:text=Our%20robot%2C%20
Maholo%2C%20conducts%20cell,cells%20and%20their%20pharmacological%20effects (last accessed March 2024)